
jumpincactus
Premium Member
Supporter
- 11,609
- 438
As a kid I always wondered why in areas that had burned due to wild fires why there was always so much lush green growth a year or 2 after a fire. Little did I know as a boy that forest fires were actually Momma Natures natural cycle of adding nutrients to the detritussphere and that it would enter eventually to the drilospehere and beyond to the rhizosphere.
When I really think about the complexity of nature and how everything is connected in the web of life I am still filled with the wonder I held as a young boy. Only difference now is instead of just guessing at how it all works there are other folks doing the legwork to help us all understand the world beneath our feet. One that is every bit as invloved and complex as the world around us and above our heads. So enough of that.
The only real question I walked away from after reading this paper was what is the correct way to determine how to dose Biochar in my garden. Enjoy!!!
P.S. The amount of images exceeds this servers limits. So here is the link where I sourced this paper so you can actually use the pictorials and graphs as it adds an enormous amount of info.
http://biocharfarms.org/farming/
Dr. David A. Laird
USDA National Soil Tilth Laboratory
Biochar not only offers a lot of environmental solutions, it can also provide the farmer and rural communities with a host of real benefits. These include improved soil quality; greater crop yields; higher fertilization efficiency; reduce contamination of groundwater from herbicides and other pollutants; heat for homes, barns, and other applications; and potentially the ability to sell carbon credits and offsets in emerging carbon markets.
If pyrolysis technologies are further developed and refined it may be possible to economically capture energy in the form of syngas and bio-oils that can be used to reduce dependence on foreign oil and gas while reducing carbon emissions.
Biochar could have an even more powerful impact on developing countries where food shortages, population pressures, and declining soil productivity often pose significant challenges. Simply put, biochar production is a long lever from which we can grab a hold of several problems turning them into opportunities to boost efficiency, economics and sustainability for the farmer.
While the potential benefits of biochar are large, there remain a lot of uncertainties and challenges. For instance, while using crop residues for biochar production is appealing, how much can be sustainably harvested without having negative impacts on soil quality? Figuring out means of sustainably harvesting, processing, and shipping biochar without expending too much money or carbon will certainly be a significant challenge. And determining the best methods of applying biochar to fields so that it is not prone to wind and water erosion is also going to be critical.
It’s prudent to stress that while biochar may have strong beneficial impacts on many soils, it’s unlikely that the dramatic increases in crop production reported for highly weathered soils in the tropics will also be shared by already fertile soils in temperate climates.
The most important challenge for biochar, in my opinion, will be integrating it into already existing models of sustainable agriculture that work to improve soil health, ecosystem services, and biodiversity. We will need to find out how biochar can work with other methods of soil carbon sequestration such as no-till, cover cropping, manuring, mulching, and agroforestry.
Biochar farming is likely to be largely experimental during the first decades. Many systems will need to be tried and tested which should offer farmers opportunities for partaking in novel and exciting research trials and a role in developing revolutionary agroecological models of “carbon farming” in which biochar plays a critical role.
BENEFITS OF BIOCHAR APPLICATIONS
Presently, agricultural is one of the most highest greenhouse gas emitting sectors of society. It’s also highly dependent on huge amounts of fossil fuels to run tractors, make fertilizer, and ship food long distances. Biochar offers a unique opportunity to help transform agriculture from being part of the problem to being part of the solution.
There are many questions that need to be addressed when it comes to biochar: What is the best method for producing biochar? What feedstock can or should be used? What is the most efficient way to capture energy from the pyrolysis process? What is the best method to apply biochar to soils and at what rates? Despite the large amount of uncertainty—including possible detrimental effects if not used in an intelligent and appropriate manner—the initial data suggest that the potential benefits of biochar to farming are significant.
Potential benefits that biochar offers for farming include:
1. Improved soil fertility and crop yields
2. Increased fertilizer efficiency use
3. Improved water retention, aeration and soil tilth
4. Higher cation exchange capacity and less nutrient runoff
5. Clean and efficient biomass energy production from crop residues and forest debris
6. Combined heat, power, and refirgeration opportunities from pyrolysis
7. Leads to net sequestration of carbon from the atmosphere to the soil thereby increasing soil organic carbon (SOC)
8. Greater on-farm profitability
9. Can be financed through carbon markets and carbon offsets
10. Decreased nitrous oxide and methane emissions from soils
11. Provides powerful tool for reversing desertification
12. Provides alternative for slash-and-burn agriculture
13. Can work as component of reforestation and aforestation efforts
14. Can produce electricity, bio-oils, and/or hydrogen fuels
15. Can use wide variety of feedstock including crop residues such as wheat and corn straw, poultry litter, cow manure, forest debris, and other farm-based biomass resources
16. Acts as a liming agent to reduce acidity of soils
Biochar is likely to have great variation over climate, soil types, and various agricultural land-use practices, and that many of these figures represent initial findings which require more research in order to substantiate. It is important to note that biochar is likely to have much greater impacts on disturbed, degraded, or high weathered soils than on soils that are high in organic matter content. For instance, a highly weathered soil in the tropics is likely to see much greater impact from biochar application than a fairly fertile soil in a temperate climate. Because most of the research that has taken place in the tropics many of the below listed findings are likely to be much greater than might be observed for the Midwest United States, for instance.
CATION EXCHANGE CAPACITY50% Increase (Glaser, 2002)
FERTILIZER EFFICIENCY 10-30% Increase (Gaunt and Cowie, 2009)
LIMING AGENT 1 Point pH Increase(Lehmann, 2006)
SOIL MOISTURE RETENTION Up to 18% Increase*(Tryon, 1948 )
CROP PRODUCTIVITY 20-120% Increase(Lehman and Rondon, 2006)
METHANE EMISSIONS100% Decrease(Rondon et al., 2005)
NITROUS OXIDE EMISSIONS50% Decrease**(Yanai, 2007; Renner, 2007)
REDUCED BULK DENSITYSoil Dependent(Laird, 2008)
MYCORRHIZAL FUNGI40% Increase***(Warnock, 2007)
BIOLOGICAL NITROGEN FIXATION50-72% Increase(Lehmann and Rondon, 2006)
*Soil moisture retnetion are mostly for sandy soils and could decrease moisture retention in clayey soils. **Nitrous oxide emission have been observed being up to 90%, but other research has found increased emissions under particular circumstances. ***Mycorrhizal fungi have been observed having up to 70% greater population levels with biochar amended soils, but have also been observed to be negatively affected by extremely high biochar application rates.
Scientific Papers:
Gaunt, J; Cowie, A. (2009) “Biochar, Greenhouse Gas Accounting and Emissions Trading” in Lehmann, J.; Joseph, S. “Biochar for Environmental Management: Science and Technology” Earthscan, Sterling, Va.
Glaser, B. Lehmann, J., Steiner, C. Nehls, T., Yousaf, M. and Zech, W. (2002) “Potential of pyrolyzed organic matter in soil amelioration”, in Proceedings of the 12thInternational Soil Conservation Conference, Beijing, China.
Lehmann, J.; John Gaunt; Marco Rondon (2006) “Bio-char Sequestration in Terrestrial Ecosystems” Mitigation and Adaptation Strategies for global Change, 11: 403-427.
BIOCHAR PHYSICAL PROPERTIES
Biochar is a light weight, highly porous material with large surface area. Surface area is important because it affects the soil’s ability to retain nutrients, water, cations, and anions. The high amount of pore space has lead some to describe biochar as an “unerground reef” material that can provide habitat for microorganisms.
BIOCHAR AND CATION EXCHANGE CAPACITY
One of the central benefits of biochar to soil quality and crop yields is its ability to retain key nutrients including nitrogen (N), phosphorus (P), potassium (K), calcium (Ca), and magnesium (Mg), along with other minor and trace nutrients. Increased plant uptake of phophorus, potasium, calcium, zinc (Zn), and copper (Cu) have been observed in the tropics.
The exact characteristics that give biochar the nutreint retention capabilities has been observed in Terra Preta but have yet to be fully explained. The answer likely lies in a combination of i) the physical structure of biochar being highly cavernous and therefore increasing pore space in soils, ii) the chemical property of having a large negatively charged surface area which increases cation exchange capacity (CEC), and iii) the promotion of greater soil organic matter (SOM) levels.
CEC is a measure of the soils ability to retain positively charged “cation” nutrients such as calcium (Ca), magnesium (Mg) potassium (K), and sodium (Na). Basically, CEC is indicates the number of negatively charged sites within a soil where cations can be housed (expressed in terms of milliequivalents of cations per 100 grams of soil).
In general, soils with higher clay contents have higher CEC, whereas soils with principal components of silt or sand have lower CECs. Addition of biochar generally increases CEC, although many studies have found that freshly produced biochar tends to have relatively low CEC and that CEC of biochar improves over period of time which can be quite rapid, perhaps as quickly as a couple of growing seasons.
The exact CEC of a biochar is primarily determined by the feedstock material used and, secondarily, by the pyrolysis conditions the feedstock is subjected to. For instance, biochar derived from poultry litter has been found to have much higher CEC than biochar derived from pine chips under the same pyrolytic conditions. CEC also appears to be maximized under higher treatment temperatures.
There is experimental research that suggests biochar may also improve nitrogen and phosphorus availability and uptake by plants–two critical plant nutrients which are not directly influenced by CEC because they are not positively charged ions. Nitrogen in the form of ammonium ions form associations with the biochar thereby decrease the possibility of nitrogen to be leached from the soil into groundwater or otherwise mineralized out of the soil.
Since nitrogen is often the most expensive and deficient nutrient in many areas of the world (and the number one greenhouse gas emissions source in agriculture), this is a critical benefit. Higher nitrgen retention properties should allow for reduced fertilizer use.
Phosophorus is another major plant nutrient which biochar seems to be able to make more available to plants. Recent warnings that phosphorus may eventually reach peak production suggest that cycling nutrients efficiently through our agricultural, composting, and waste treatment facilities will be vital and that biochar may assist in the process.
In sum, the observation data gathered so far indicates that biochar may have a powerful role in retaining nutrients and thereby increasing nutrient bioavailability, though considerably more observational data is needed.
BIOCHAR AND FERTILIZATION EFFICIENCY
Greater nutrient retention implies that less fertilizers need to be applied to achieve a given crop yield. This is particularly important for nitrogen fertilizer which often constitutes that largest agricultural input with some 207 million metric tons applied in the 2007-2008 growing season globally (FAO, 2008.)
Modeling future demand for nitrogen fertilizer, the Food and Agriculture Organization has predicted that demand will outstrip supply in 2011 at some 240 million metric tons. Further, nitrogen fertilizer use is a significant contributor to global climate change as nitrous oxide (N2O), which is approximately 300 times as powerful a greenhouse gas than carbon dioxide (CO2), is often volatilized into the atmosphere at massive rates.
Because the vast majority of nitrogen fertilizer is derived from natural gas (CH4) via the Haber-Bosch process, the price of nitrogen fertilizer is directly subject to the rises and falls in energy market which although unpredicitable in the short-term are likely to rise under long-term time horizons.
Gaunt and Cowie (2009), in an assessment of biochar’s ability to reduce greenhouse gases, have estimated a 10-30% reduction of nitrogen fertilizer use. Use of biochar has also been observed to reduce emissions of N2O directly from soils by 15-90% (Yanai et al. 2007; Renner, 2007), although one study (Yanai et al. 2007) also found a greater N2O emissions under one set of conditions.
Interestingly, Sohi et al. (2009) have suggested the concept of using syngas from the pyrolysis process to replace natural gas (CH4, methane) to produce nitrogen which could be then be applied to biochar that is produced in the same process to create a powerful carbon and nitrogen rich fertilizer based on the research done by Day et al. (2005). Small scale nitrogen fertilizer plants have been proposed for Africa in order to boost soil fertility and crop production; perhaps it is time we start to consider running these plants using pyrolysis technologies which would vastly increase the sustainability and lower the greenhouse gas emissions associated with producing and applying these nitrogen fertilizers. Below I also examine how anaerobic digester sludge and human urine–both rich in nitrogen–could also be used to charge biochar with nutrients. Use of nitrogen fixing leguminous trees and cover crops in tandem with biochar applications should also be explored.
Scientific Papers:
Day, D., Evans, R.J., Lee, J.W. and Reicosky, D. (2005) “Economical CO2, SOx , and NOx capture from fossil-fuel utilization with combined renewable hydrogen production and large-scale carbon sequestration,” Energy 30, 2558–2579.
Sohi, Saran, Elisa Lopez-Capel, Evelyn Krull, Roland Bol. “Biochar, climate change, and soil: A review to guide future research,” CSIRO 1834-6618, 2009.
Yanai, Y. Toyota, K. and Okazaki, M. (2007) “Effects of charcoal addition on N₂O emissions from soil resulting from rewetting air-dried soil in short-term laboratory experiments”, Soil Science and Plant Nutrition, vol. 53, pp181-188.
BIOCHAR APPLICATION
Many people are interested in applying biochar to their farm or garden which can provide an excellent opportunity for experimenting with and observing the impacts of biochar on crop productivity and soil quality.
The first step is sourcing the biochar material. There are essentially two options: make the charcoal yourself (described later in this section), or buy the charcoal from a company that produces biochar.
The second step is determining the best application methods for your specific area and determining the appropriate rates. “Charging” the biochar with nutrients (such as compost, soluble inorganic fertilizer, or other nutrient sources) has been recommended by many.
An important issue that must be considered is whether biochar should be incorporated using mechanical tillage. Tillage can lead to the loss of large amounts of soil carbon as aeration tends to encourage microbial decomposition of organic matter and subsequent mineralization of soil carbon stocks. A field that has been under no-till management can loss somewhere on the order of 5 or 10 years of carbon accumulation with a single year of tillage. Thus, incorporation of biochar using tillage is counterproductive to our overall goal of soil carbon sequestration. But what if tillage is the only way in some management systems to get the biochar into the ground? Most biochars are likely to be highly susceptible to erosional processes as it is both light (it has a bulk density of 0.17-0.37 tons per cubic meter) and is often hydrophobic when freshly produced. Thus, biochar is extremely prone to runoff from heavy storms with its high buoyancy and it’s also extremely prone to wind erosion due to its light nature and to the fact that biochar tends to have a high dust fraction that can be easily made airborne during transport, incorporation, and post-application.
Because biochar is light and can often contain large dust fractions care must be taken to reduce losses of biochar from the field. The above photo shows loss of biochar during transport. From BlueLeaf Inc., Quebec, Canada.
Major et al. (2009), for instance, found significant loss of biochar (approximately 50%) when applied to tropical soils. Thus, some form of incorporation seems to be necessary. The question is whether and what specific tillage systems are the best or most effective means of incorporation biochar while trying to minimize soil distrubance and loss of soil organic matter and carbon.
Blackwell et al. (2009) provide an excellent description of possible biochar application methods (in “Biochar for Environmental Management” edited by Lehmann and Joseph) including: addition to solid compost with subsequent application via manure spreader; addition of biochar to manure slurry with subsequent spraying onto or injection into soils; pelletization with manure, flour, and water that can be broadcasted onto field; deep banding of biochar using pneumatic systems or incorporation into trenches; and, lastly, top-dressing biochar where biochar is simply spread onto the soil surface without any direct incorporation.
Work must be done to engineer and implement the most efficient and sustainable means of applying biochar for different agricultural systems. At the garden level, incorporation of biochar into a garden plot or raised beds should be a fairly simple task. At the farm scale several alternative tillage systems that may be of interest include the Keyline Plow, organic no-till using a Crop Roller, and the Rotocult.
The Keyline Plow:
The Crop Roller:
Scientific Papers:
Blackwell, P.; Riethmuller, G.; Collins, M. (2009) “Biochar Application to Soil,” in in Lehmann, J.; Joseph, S. (2009) “Biochar for Environmental Management: Science and Technology” Earthscan, Sterling, Va.
Major, J.; Lehmann, J.; Rondon, M.; Goodale, C. (2009) “Fate of Soil Applied Black Carbon,” Global Change Biology.
DOCUMENTING YOUR BIOCHAR EXPERIMENT
I highly recommend to consider running the biochar application as close as possible as a scientific experiment. This can be done by by taking pictures, recording all the pertinent information (e.g. feedstock material and source of biochar, application rate, method of application, crops or pasture grown, any auxiliary fertilizers used, etc.) and documenting the results as carefully as possible. The International Biochar Initiative (IBI) has published a “Guide to Conducting Biochar Trials” by Dr. Julie Major which provides an excellent overview of the steps and procedures for conducting a biochar experiment.
It may even be worthwhile to contact a local university, college, agriculture extension office, or high school in order to get involvement by a member of the scientific community who can assist in conducting a more thorough scientific experiment. The results should be interesting and the process fun. An online database has been established to document biochar trials on the International Biochar Initiative website (contact Dr. Julie Major).
BIOCHAR AND WATER RETENTION
The ability of biochar to improve soil water retention is important in light of global climate change as changing precipitation patterns are predicted with increasing global temperatures. In many areas of the world it is expected that less frequent but more intense storms are likely to become prevalent. This could hurt agricultural production not only because it will likely increase the number of dry days without any precipitation, but when the precipitation does come it will come in more intense storms which risk increasing the erosion of soils. Biochar may have the ability to mitigate drought by increasing soil moisture, while decreasing soil erosion and nutrient leaching. Thus, Micronesia has formally recommended that biochar be considered as a “clean development mechanism” (CDM) within the Kyoto Protocol through the United Nations Convention on Combating Desertification (UNCCD.)
Increased moisture retention and water bioavailability is thought to be a critical factor for greater yields with biochar amended fields. Generally, water retention is increased as the rate of soil organic matter increases. Sohi et al. (2009) have hypothesized that additions of biochar may lead to increased levels of soil organic matter which may partially explain improved water retention, and Glaser et al. (2002) found that Terra Preta soils rich in charcoal had 18% greater water retention than neighboring soils that did not have significant charcoal deposits.
Having a highly porous structure biochar is also thought to improve soil porosity particularly in sandy soils.
Importantly, low temperature pyrolysis biochar have been show to have initial hydrophobic (water avoiding) properties. Thus, research is needed to determine the properties of biochars created by various processes which are important to determine the degree to which they may be able to aid in greater soil water retention and how this property may change over time. For instance, it may be more adventageous to add medium-to-higher temperature pyrolysis biochars to soils that are particularly vulnerable to low soil moisture rates since these biochar are likely to better at retaining soil moisture.
THE SOIL FOOD WEB
The “soil food web” refers to the complex interaction between biological communities of plants, bacteria, protozoa, fungi, nematodes and larger “macro-organisms” which, in part, drive dynamic soil processes. Each of these biological components provides ecosystem services including decomposition, nutrient cycling, filtration and soil formation.
Because biochar influences both physical and chemical properties of soils, it can have a significant effect on the various biological communities. Research on how biochar may alter biological processes in soil is limited, but suggests that biochar could boost population levels of beneficial fungi and bacteria thereby increasing soil health and plant productivity. It has been suggested that biochar with its highly porous exoskeleton is akin to an underground coral reef that could provide habitat, nourishment, and protection to various biological communities in the
MYCORRHIZAL FUNGI
Recent studies suggest that biochar may, under certain circumstances, have a positive affect on the occurence of mycorrhizal fungi populations and their association with plant root systems. Because mycorrhizal fungi are critical and ubiquitous components of terrestrial ecosystems that can have dramatic beneficial effects on plant productivity, there is significant interest in the effect of biochar on this special soil fungi.
Mycorrhizal fungi is an important but often overlooked aspect of soil plant production and soil formation. Mycorrhizal fungi establish mutualistic relationship with the plants. Most often this relationship is symbiotic—both the plant and fungi benefit from one another—but can also be parasitic—the fungi benefits at the detriment of the plan—where extremely low levels of phosphorus or other nutrients are available.
The exchange is basically this: the plant provides energy rich carbohydrates made from photosynthesis to the fungi which the fungi could not otherwise obtain. In exchange the fungi shoots forth a web of hyphae that extend the reach of the plant’s roots and funnel water and nutrients from the soil to the plant. The nutrients delivered to the plants are typically phosphorus, copper, and zinc—the most important being phosphorus. The extent of this relationship is vast with approximately 90% of all terrestrial land plants having associations with mycorrhizal fungi and 66% of terrestrial land plants having associations with the most common soil fungi, arbuscular mycorrhizal fungi (AMF).
Research by Daniel Warnock (see “Mycorrhizal Responses to Biochar in Soils”; Warnock et al., 2007) indicates that the colonization rate of arbuscular mycorrhizal fungi may increase significantly with biochar applications, although later studies using non-herbaceous biochar showed the opposite results (Warnock et al., 2008), therefore highlighting the importance of choosing biochar feedstock carefully and the need for further study to determine the characteristics of biochars from a wide variety of feedstocks produced under multiple pyrolysis procedures. Nishio and Okano (1991) found that when 1 kilogram per square meter of biochar was added to volcanic ash soil that alfalfa associations with AMF increased by 40-80% in Japan.
In 1996, Dr. Sarah F. Wright and PhD student Kristine Nichols, researchers at USDA Agricultural Research Services (ARS), discovered a substance in the soil she later dubbed “glomalin,” a significant and widespread soil carbon source that had previously been completely overlooked. The importance of glomalin has yet to be fully established, however, four characteristics reported by Wright and Nichols make it particularly intriguing.
First, glomalin is pervasive—it is found in practically all soils across the world, though in different magnitudes (more prevalent in temperate regions and less so in tropical areas.) Second, glomalin provides a huge carbon store—roughly roughly 27% of total soil carbon according to ARS research. Third, glomalin lasts a long time in soils—it can survive between 7 and 42 years. And, lastly, it’s sticky—it plays an important role in aggregating and binding together sand, clay, and silt particles which is a precursor to soil formation.
Microscopic image of arbuscular mycorrhizal associations with corn roots; the substance covering the AMF is glomalin thought to be a major source of soil carbon (USDA Agricultural Research Service)
Interestingly, arbuscular mycorrhizal fungi appear to be the only producers of glomalin which occur in abundance on it’s spores and hyphae. Glomalin is a type of glycoprotein (proteins composed of polypeptide and oligosaccharide chains), containing 30 to 40% carbon. Thus glomalin may account for roughly 4 times the amount of total carbon in soil than does humic acid. Wright, speculating on the reason why abuscular mycorrhizal fungi produces glomalin says that, “We’ve seen glomalin on the outside of hyphae, and we believe this is how the hypahae seal themselves so they can carry water and nutrients. It may also be what gives them the rigidity they need to span the air spaces between soil particles.” Wright has speculated that this may partially account for the “missing carbon sink” (see“Glomalin: Hiding Place for One-Third the World’s Carbon”).
Some have speculated that the regenerative nature of glomalin to directly sequester atmospheric carbon from the atmosphere fixing it into the soil may partially explain the “regenerative” nature of ancient Terra Preta soils, though this remains an interesting hypothesis at present with little to no confirmation. Clearly, more research is needed to assess the role of biochar may have on mycorrhizal levels in different soils using various types of biochars.
ARE ALL BIOCHARS THE SAME?
One thing that can be definitively stated about biochar is that they are not all the same. The properties and characteristics (and therefore the agronomic quality) of a particular biochar depends upon a number of interacting feedstock and process variables including the pyrolysis type (slow, fast, intermediate, steam gasification, microwave, etc.); the residence time (fast, slow, intermediate); the temperature (too hot, too cold, just right!); the feedstock material used (all plant material vs. inclusion of manure other high nutrient waste); and whether any additional nutrients were added to the biochar prior to application.
Biochars created from plant materials tend to be lower in nutrient content than biochar created from poultry litter or other manure waste products (Lehmann et al., 2003). One study by Chan et al. (2008) found little to no positive effects to plant growth when a low-nitrogen biochar created solely from plant materials was applied to the soils, but when biochars made from poultry litter (very high in both nitrogen and phosphorus) were applied positive impacts were observed.
(From "A Handful of Carbon" by Johannes Lehmann published in Nature Magazine, May 2007)
All this begs the question, “How is the best biochar made?” “Is there a best pyrolysis method?” “Are there a certain feedstock which are more optimal than others? ” “Is there a specific temperature and residence time at which the biochar obtains optimal properties?” “Is there a certain nutrient rich substance which could best “charge” the biochar prior to application?” We obviously do not have the necessary data to be able to provide answers to all these question. One thing we can be fairly certain of, however, is that the answer to these questions is going to depend the geography, climate, soil types, available materials and desired outcomes of a particular place.
“DESIGNER” BIOCHAR
One might imagine there being a “sweet spot,” so to speak, for pyrolysis production for a region. For instance, if we were in a semi-arid climate we would definitely not want our biochar to have any sort of hydrophobic tendency and therefore might have to stay away from low-temperature pyrolysis. Indeed, we would likely opt for the biochar that delivered the very best water retention qualities possible. We could experiment with the available biomass feedstocks, testing each under a variety of pyrolysis technologies–slow, fast, steam gasification, microwave, etc.–and at different temperature ranges to find which one delivered the very best moisture retention characteristics. In another region, say one with already fairly rich soils, we may not care about the end biochar product nearly as maximizing energy production while simultaneously capturing a certain percentage of carbon as biochar for carbon credits.
Changing properties of biochar from black locust feedstock as a function of temperature. From Lehmann et al., 2007.
The point of the above example is to demonstrate a) not all biochars are the same, and b) we may be able to use this to our advantage to optimze our production to fit our needs. The most salient point, however, remains that more research is necessary. Hence in a recent publication by Chan et al. (2008), it was stated that, “Despite the recent interest interest in biochars as soil amendments for improving soil quality and increasing soil carbon sequestration, there is inadequate knowledge on the soil amendment properties of these materials produced from different feedstocks and under different pyrolysis conditions.”
Scientific Papers:
Chan, K.Y., Xu, Z. (2009) “Biochar: nutrient Properties and Their Enhancement” in Lehmann, J.; Joseph, S. (2009) “Biochar for Environmental Management: Science and Technology” Earthscan, Sterling, Va.
Chan, K.Y., Van Zwieten, L., Meszaros, I., Downie, A. and Joseph, S. (2007b) “Assessing the agronomic values of constrasting char materials on Australian hardsetting soil”, in Proceedings of the Conference of the International Agrichar Initiative, 30 April-2 May 2007, Terrigal, NSW, Australia.
Novak, J. et al. USDA Agricultural Research Services ongoing research on “Designer Biochars”.
HEALTH AND SAFETY CONCERNS
There are several health and safety issues associated with using biochar including 1) inhalation of charcoal dust during transport and application, 2) safety issues of storing and transporting charcoal mainly from the risk of combustion, 3) introduction of harmful substances into the soil from contaiminated biochar, and 4) use of dangerous and toxic chemicals after biochar application that are adsorped by the charcoal.
A fine charcoal dust often accumulates even when creating large biochar lumps. This can create problems during storage, transport and application as the dust has a tendency to become airborne thereby causing both health issues from inhalation of the dust and safety issues eminating from the possibility that the dust could ignite. Despite the widespread belief that wet charcoal presents safety problems, wetting the biochar will ameliorate health and safety issues eminating from charcoal dust. (If the moistening agent simultaneously charged the biochar with nutrients, this may be an opportunity for accomplishing two tasks at once.) For more information on charcoal handling and storage, see the FAO’s primer on the topic.
One of the principal concerns is the introduction of harmful substances or toxins into the soil. The process of pyrolysis has been found to create both polycylic aromatic hydrocarbons (PAHs) as well as dioxins–both of which are fairly nasty toxins–which may be introduced into the soil with applications of biochar. Intitial studies, however, have found the levels of these potential toxins to be below the safe threshold level.
Sohi et al. (2009) have written that, “Analysis of a limited number of biochar samples has indicated concentrations of toxic combustion products such as polycyclic aromatic hydrocarbons that are not at environmental risk level. However, a more systematic evaluation for a more complete range of other potentially harmful chemical contaminants associated with combustion, as well as toxic substances within feedstocks, has not been made.”
Visual representation of polycycilic aromatic hydrocarbon molecular structure (Wikipedia)
Analyzing fast pyrolysis technologies, Garcia-Perez (2008) found that “PAH production levels are significantly reduced at lower temperatures (below 700°C). In addition, dioxin production is significantly reduced when chlorine and metals are missing.” Gaunt and Lehmann (2008) reported that, “the process temperature parameters under slow pyrolysis are such that we avoid the formation of polyaromatic hydrocarbons in the biochar product.”
Only a handful of studies are available on the issue of PAHs formed during pyrolytic reactions. Sohi et al. (2009) reported that that in personal communication with Dr. David Manning, a Geologist at the University of Newcastle, that recent studies have suggested that the levels of PAHs in biochars are practically the same for most soils. Another analysis by Brown et al. (2006) found PAH concentrations of biochars (3-16 micrograms per gram of biochar) to be lower than that of a controlled burning done within a pine forest (28 micrograms per gram of char).
In order to reduce the risk of producing dioxins and to prevent contaimination of other varieties such as heavy metals precaution should be urged for use of feedstock materials. Sewage sludge, for instance, can be pyrolyzed; however, it would behoove us to abstain from applying the resulting biochar to crops destined for human consumption due to presence of high levels of potentially harmful substances.Thepotential presence of PAHs and dioxins is, in my opinion, the most pernicious issue facing widespread adoption today. A careful, unbaised, and critical examination of this issue is necessary.
Another question that must be addressed is the buildup of secondary compounds. Research has pointed to biochar as being a means to prevent groundwater pollution by capturing fertilizers that lead to eutrophication of waterways as well as pesticides, fungicides, and herbicides such as “atrazine.” (See this recent study by Cao et al. (2009) which documents the ability of biochar derived from dairy manure to trap organic pollutants and heavy metals such as atrazine and lead.)
The ability to capture harmful chemical compounds is typically discussed as a beneficial affect of biochar application, but there remains the question of whether the ability of biochar to capture these compounds may lead to other negative health and safety implications. An novel means of dealing with hydrocarbon compounds has been reported by mycologist Paul Stamets. An interesting research question to test would be whether innoculation of biochar compost piles with fungi such as oyster mushrooms may be able to effectively destroy PAH compounds.
BIOCHAR APPLICATION RATES
The amount of biochar that should be applied to the soil is still an area of active research. Many studies point to 50-60 tons per hectare (20-25 tons per acre) as being an optimal rate of biochar production, however this is a very large amount of material to apply. The objective for biochar farming is probably not going to be applying as much biochar as possible, but rather to achieve the maximize crop production from the most efficient application of biochar. Dr. Johannes Lehmann has reported that, “With relatively small amounts of 2-5 Mg C per hectare [tones/hectare] of bio-char, significant improvements of crop growth can be observed.”
Increase in plant biomass production as a function of amount of biochar applied. From Lehmann and Rondon, 2007.
Thus, the grower would want to know where there area of maximal efficiency lies, which will change according to crop, climate, soil type, etc. This is question of bioregionally appropriate biochar application is an excellent research question for agricultural extension agencies. In addition to trial plots to determine the actual effects, the use of “decision theory” probabilistic modeling would be an appropriate approach to answering this question.
Invariably it is going to be many years—probably decades—before the exact recommended quantities of biochar are worked out for specific crops, for specific soil types, and for specific climates. This is also represents an area that early adopters of biochar farming could greatly benefit the rest of the farming community and the world by converting several acres of their farm to experimental biochar additions. Careful application, observation, and record keeping by farmers–while not necessarily “publishable” in scientific journals–may provide incredibly important initial evidence for the likely effects of biochar in different environments and under a wide variety of conditions.
PURCHASING BIOCHAR
There are a number of companies which have formed recently that are now marketing or selling biochar. The following companies are those which I am aware of that are selling biochar directly to the general public for agricultural uses. Many of them are able to ship amounts ranging from 10 pounds for garden use all the way up to many tons for the larger farm.
A recommendation is that you ask what the process that was used to make the biochar (i.e. slow pyrolysis, fast pyrolysis, or gasification) and what type of feedstock material was used to create the biochar. I would also request to see a copy of a lab analysis which provides information on the contents and characteristics of the biochar including major and minor nutrients, pH, moisture content, and presence of volatiles. It is also a good idea to request that a MSDS (Materials Safety Data Sheet) be provided prior to shipment.
The following is a listing of companies that I am aware of which are presently selling biochar (or natural charcoal without additives marketed for other purposes).
BIOCHAR SUPPLIERS
“CharGrow” from CarbonChar
Requires a minimum of four pallets with each containing 40 50-pound bags (not exactly feasible for anyone except the large land owner.) Based out of New Jersey, US.
“Agri-Char” from BEST Energies
One of the most well established slow pyrolysis companies. Website contains no specific information regarding purchasing of biochar, but may be able to purchase by directly contacting. Based out of Madison, Wisconsin, US and with a test facility in Somersby, Australia.
“EternaGreen™ Biochar” from Biochar Brokers
Biochar produced from woody biomass feedstock; can take larger orders of more than a ton . Based out of Pennsylvania, Colorado, and Tennessee.
“Biochar Plus” from the Biochar Engineering Company
$10US for 10 kg bag of biochar plus . Based out of Golden, Colorado, US.
“Fine Horticulture Charcoal” from Quarter Acres Orchards
“Gallon Bag” (sic) for $6.50 US; I am not knowledgeable whether “carbon negative” pyrolysis is being used to make this charcoal. Based out of Kensington, Maryland.
“Horticultural Charcoal” from Black Gold2 quart bags (sic) available. Sourced from “Western, United States.”
Alibaba Product Search for “Agricultural Charcoal”
Many companies listed from around the world. The sustainability of the companies listed on this site is unknown as are charcoal production methods.
“Hardlump Charcoal” by Cowboy Charcoal Company
A natural hardwood charcoal marketed for barbecuing which can be used as a soil amendment. Claim to have “state-of-the art environmentally compatible wood retort plant.” Based out of Albany, Kentucky.
“Granular Horticulture Charcoal” by Lazarri
Sell several different sizes of charcoal (lump, granular, and powder) from hardwood feedstock coming in 20, 40 and 50 lbs. bags. Based out of Brisbane, California.
“CQuest Biochar” from Dynamotive
Produced in a fast-pyrolysis process with “EcoLogo” Certification; appears to be marketed more as a fuel than a soil amendment; unknown feedstock material; potentially high volatile matter (VM) content. Based out of West Lorne, Ontario, CA.
Various Wood Type Charcoals from Real Montana Charcoal
Sell a wide variety of charcoal from different wood types (apple, maple, plum, oak, etc.); uncertain about sustainability of charring process. Approximately $1/pound for most types. Based out of Florence, Montana, US.
Having listed the following companies from whom you can purchase biochar, I wanted to comment on the issue of shipping biochar long distances: It doesn’t make sense. There’s only a certain range at which biochar can be transported for it 1) to be economical, and 2) to sequester more carbon than is required to make and apply it. There’s a critical threshold distance at which the fact that petroleum is being used to transport the biochar drives the process from being carbon negative to being neutral and then immediately to carbon positive.
I have yet to come across a study which establishes this transportation threshold. The distance is likely to vary somewhat place-to-place, but should be fairly universal within shipping methods (e.g. 18-wheeler tractor trailer, cargo ship, etc.) Fortunately, most areas have ample supplies of biomass materials from which biochar can be produced. The upshot of the fact that biochar can only be economical and sustainable within certain geographic/spatial scales and that in most areas biochar is readily available, is that biochar production probably lends itself toward a smaller-scale, decentralized approach. Fortunately, this is likely to be beneficial in terms of job creation potential, development of local heat and power infrastructure, and promotion of small-to-medium scale operations.
BIOCHAR PRODUCTION FOR GARDEN OR FARM
One of the key benefits of biochar is that it can produced on an extremely small scale although a carefully designed system is necessary to insure that dangerous off-gases are not relased into the atmosphere. For the homegardener there are many designs and descriptions of inexpensive and small-scale methods for making biochar that have been published on the internet. Many of these are based on the use of 55-gallon drums used as retort kilns. The use of the 55-gallon drum retort benefits from its simplicity, inexpensiveness, and ability to produce biochar for the homegarden scale.
A double barrel retort kiln design (Courtesy Twin Oaks Forge)
A serious potential downside of simple charcoal kilns, however, is that they can produce a host of detrimental emissions including CO, NOx and SOx as well as powerful greenhouse gases including methane (CH4) and nitrous oxide (N2O). More elegant 55-gallon retort designs will destroy these gases in a secondary combustion process. If these gases are not combusted the net emissions of biochar production can be detrimental for the climate system (as opposed to being beneficial). A gooddescription of making an effective 55 gallon drum retort that destroys off-gasescan be found on Mr. Folke Gunther’s website. Another 55-gallon drum system I like quite well, pictured above, makes use of two drums which combust the gases directly below the barrels.
A drawback of the 55-gallon drum kiln is that they are not able to produce biochar in the quantity to supply the farm scale. Further, most 55-gallon drum retorts fail to capture or otherwise make use of excess energy produced during the reaction. If there are any heat applications which could be taken advantage of, this would allow for a greater return on the process, hopefully replacing fossil fuel use in the process. For instance, if a heat exchanger were incorporated into the above design design and waste heat was transfered to an insulated hot water tank, the waste heat could be utilized to heat a building space (e.g. greenhouse or poultry barn) with a hydronic steam or hot water system.
Other small-scale farm designs exist. The development of small-scale, high-efficiency pyrolysis cookstoves (such as those being developed by Mr. Robert Flanagan and the WorldStove Company) cleanly produce biochar from crop waste while providing heat for cooking. Development and deployment of these cookstoves could have important implications for least developed countries (LDCs). A 5,000 UK pound ($7,000 US dollar) grant was recently awarded to The Rainforest Information Centre by The Artists Project Planet in order to develop a low-cost, open-source pyrolysis kiln that could be used in developing countries.
A mobile pyrolysis unit well suited for farm-scale use (Courtesy Agri-Therm)
Agri-Therm LLC, based out of Ontario, Canada, is presently working on developing a commercially available fully-automated mobile pyrolysis unit that can process between 2 and 40 tons of biomass per day. Seeing that crop residues and other feedstocks may only be available seasonally it is likely that farmers would only need access to a pyrolysis plant during a portion of the entire year. One could imagine a group of farmers co-investing in one such unit to be used among them instead of having to solely invest in a non-mobile unit.
NOTE ON USING CHARCOAL BRIQUETTES
Briquette charcoals are those which are manufactured to have a uniform size, appearance, and combustion properties. In order to achieve this uniformity a variety of additives are used . These often include starches or clays (as binding agents), sodium or potassium nitrate (to speed up ignition), anthracite coal (to provide longer burn-time), pulverized limestone, and sodium borate (i.e. “borax,” to increase burn time.)
While some of these products (starches, clays, and limestone) would probably be quite benign or even possibly slightly positive to soil quality, the rest would likely be fairly detrimental.
I would recommend against using charcoal briquettes as they contain additives that may be harmful to plants
According to a University of Hawaii study, processed charcoal briquettes can have Volatile Matter (VM) levels as high as 36.4% which could actually decrease plant productivity. Acceptable levels, according to this study, are those below ~7 or 8% VM. In short, I would tend to recommend against using any sort of processed briquette product.
“CHARGING” OF BIOCHAR
After you have acquired the biochar, it is highly recommended that you “charge” the biochar with nutrients before you apply it. This is important: “raw” biochar (straight from the kiln without any nutrient charging) will likely have neutral or even slightly negative effects during the initial phase. This is due to the fact that biochar is very good at holding on to nutrients.
Biochar has a very high cation exchange capacity or CEC and is therefore able to hold onto positively charged nutrients such as calcium (Ca), magnesium (Mg) potassium (K), and sodium (Na), and is also very good at holding onto nitrogen (N) and phosphorus (P). If applied raw to soils nutrients will bond to the biochar thereby making them unavailable for the plant to use (i.e. the “bioavailability” of the nutrients has decreased though the nutrients themselves still remain in the soil.)
If one were to add raw biochar to the soil the long term effect would still be positive, but this would require time for the biochar to “soak up” nutrients until most or all of the pores and negative charges are saturated with nutrients. Then the biochar would act as a slow release fertilizer that efficiently releases a steady stream of nutrients.
Dr. David Laird, of the USDA National Tilth Laboratory, describes biochar as “a fantastic adsorbent and when present in soils it increases the soil’s capacity to adsorb plant nutrients … charcoal contains most of the plant nutrients that were removed when the biomass was harvested and has the capacity to slowly release those nutrients to growing plants,” in a paper on biochar called “The Charcoal Vision“.
Close-up of biochar soil aggregates and mycorrhizal fungal associations on plant roots (Richard Haard)
By pre-charging the biochar with nutrients prior to application we can greatly speed up this absorption process and thereby mitigate any negative effects the biochar may initially have as it tries to satisfy its craving for filling nutrients. Sources of nutrients that can be used with biochar include compost, manure, poultry litter, human urine, and inorganic fertilizers. (This is discussed in more detail below.)
BIOCHAR COMPOSTING
Composting of organic materials is an essential way to cycle nutrients back into the soil especially on organic farms or in areas that don’t have access to chemically derived fertilizers. It then comes at little surprise that Eliot Coleman has said that, “Producing quality compost is the most important job on the organic farm. A lot of the problems I see on farms I visit could be solved by making better compost.” High levels of soil organic matter (SOM) that come from addition of compost, manure, cover crops, and other organic soil builders also decreases the risk of drought by retaining more soil moisture. According to one study by the Rodale Institute, organic fields can often outperform conventional fields by 35-100% during periods of severe drought.
Incorporating biochar into an existing composting system–whether at the garden scale or the farm scale–should not be too difficult and may help retain nutrients that would otherwise be volatilized out of the compost, nitrogen in particular which can escape as ammonia (NH3) gas. Biochar may also likely assist in moisture retention of organic waste piles and increase microbial populations thereby increasing the rate at which materials are broken down into friable compost.
Biochar composting can minimize carbon and nitrogen mineralization and volitalization (Barry Batchelor, Terra Preta List)
Critical to composting is maintaining a balance between carbon (often referred to as “brown” organic materials with high carbon to nitrogen ratios generally above 100:1 such as corn husks, saw dust, and woody mulch) and nitrogen (“green” organic materials with C:N ratios less than 100:1 such as grass clippings, kitchen waste, and leaves) For home gardeners a layering approach of adding consecutive piles of green and brown. “Biodynamics” agricultural practioners often use complex methodologies for creating rich compost involving the addition of rock powders, kelp, and microbial innoculants which I think may provide a nice framework to developing a biochar composting system. For instance, see the following description of making compost. I would imagine including a thick layer of biochar as the foundation of the compost pile would avoid nutrients being leached out, while incorporating thin layers of biochar within the green and brown layers would prevent nutrient volitilization. (More information below on creating “Terra Preta Nova”).
Biochar could be easily incorporated into farm-scale systems such as windrow composting (Virginia Tech Extension Office)
At the farm or muncipal scale biochar could be also be of benefit to composting. The use of biochar within hog slurry retention ponds, windrow composting systems, and manure piles seems quite feasible having the same benefits of reducing the rate of nutrient leaching and volatlization. Use of biochar within poultry production as an addative to the bedding layer also holds a lot of promise (more on that below). I have yet to read any studies of use of biochar within these types of composting systems but there is strong reason to believe that there would be multiple beneficial effects.
BIOCHAR ECOSANITATION
Some may find the idea of using human urine as a fertilizer a disturbing concept. Despite generally being thought of as waste, the fact is that urine is nearly sterile and contains a highly concentrated and relatively well-balanced range of major nutrients with a N:p:K ratio of 18:2:5 as well as a host of minor and micro-nutrients. Using urine as fertilizer could provide a major source of nitrogen particularly for developing countries in which chemically derived nitrogen fertilizers are simply too expensive or altogether unavailable as is the case for much of Africa. The average human excretes approximately 1-2 liters per day, equating to roughly 10 billion liters per day over the entire world or 3.6 trillion liters per year. If , for the sake of argument, one-tenth of this urine were to be effectively captured for use as a nitrogen fertilizer this would equate to some 64 million metric tons (70 million standard tons) thereby offsetting 30% of global nitrogen demand.
Picture of a urine diverting toilet which allows for the separation of dry and liquid human waste
Use of a simple urine diverting toilet, such as the one pictured above, allows for the separation of dry and liquid human waste. Thus urine could be collected, stored and used to charge biochar prior to application.
One of the reasons why urine makes a good fertilizer is that most of it is directly available to plants. Both the potassium (K) and the phosphorus (P) are found in inorganic forms which are readily uptaken by plants. In terms of the nitrogen (N) content, roughly 10-30% of urine is in the form of ammonia and creatine, the remainder being 70-90% in the form of urea. The urea is fairly rapidly decomposition by urease into ammonia and water constituents and subject to volatilization and evaporation. One of the qualities of biochar, however, is to be able to latch onto nitrogen. I have not read any studies on the combined use of biochar and nitrogen, but feel this may be an extremely fruitful area to study in terms of developing sustainable nutrient cycling and fertility programs.
In a 2007 study in Findland, Dr. S. K. Pradhan found that the use of human urine lead to a net increase of 50% more biomass than without urine. Interestingly, it also generated a greater than 9% increase over conventional fertilizers despite the fact that the conventional fertilizers had an N:p:K ratio significantly higher (10.2: 6.8: 19.2) than the urine (10.2: 07: 3.9).
Composting toilets which safely breakdown human feces over a period of time thereby leading to the destruction of pathogens is also recommended. Most manufacturers of composting toilets recommend adding a high carbon material such as peat, sawdust, or wood chips to absorb moisture and prevent odor. Instead, might it be possible and indeed more beneficial to add biochar instead?
Two-part composting toilet that could be used to cycle nutrients in human waste (EconciousMarket)
Being able to cycle nutrients back to the soil is critical for sustainable management of agricultural lands. This is especially the case as we consider the i) the greenhouse gases associated with nitrogen fertilizer manufacturing (which is almost exclusively made from natural gas presently) ii) application of ammonia fertilizer can lead to volatilization of nitrogen in the form of N2O which is 310 times more powerful than CO2 as a greenhouse gas, and iii) fertilizers are likely to become more expensive with the rising cost of natural gas and the declining availability of phosphorus, iv) many communities and municipalities spend upwards of 25% of their energy on sewage treatment, and v) freshwater becomes increasingly scarce (toilets use approximately 30% of household water use.)
This bring new light to the musing Theodore Roosevelt once had that, “Civilized people ought to know how to dispose of the sewage in some better way than putting it in the drinking water.”
TREATMENT WITH ANAEROBIC DIGESTER SLUDGE
Another option for nutrient charging biochar could be done using the byproduct of another energy product system–anaerobic digester sludge. While pyrolysis produces a carbon-rich material that really should be charged with nutrients prior to application, anaerobic digesters produce a nitrogen-rich sludge ideal for charging biochar with nutrients. Further, anaerobic digesters also produce a large stream of methane which could used to fire the initial endothermic stages of slow pyrolysis. Thus biochar production and methane digesters may be well suited to work hand-in-hand.
The Pura Village Community Biogas Project offers a case study and published datafor considering the use of anaerobic digesters with pyrolysis technologies. The project was essentially a cooperative effort in the early 1980’s on behalf of local university researchers, NGOs, and the community of Pura, in Karnataka, India to build a biogas plant to replace wood biomass as their primary fuel for cooking. The sole inputs into the system were water and cow dung. Later the biogas production was fed into a generation system to produce electricity for lighting. Two “floating-drum” digesters were constructed along with a distribution system to provide access to 56 families in the village. Description of a small-scale biogas digester system can be found here.
Anaerobic digesters can range from complex large-scale continuous feed systems to small scale systems constructed of steel drums such as the floating-drum system pictured here (Charlie Forst, 2001)
Based on the performance of the system, the daily input for a ~42.5 m^3 biogas (approximately 60% CH4 and 40% CO2) is 1.25 tons of cattle dung mixed with 1.25 m^3 water per day. The resulting daily output, in addition to the 42.5 m^3 biogas, is approximately 2.45 m^3 of digested slurry containing 3.6 kg of nitrogen (2.2% of the total). The total amount of nitrogen coming out of the biogas system was equal to the amount that was put in—thus, nitrogen is conserved.
Pyrolysis systems could be coupled to biogas systems in a number of ways. First, the nitrogen rich sludge could be directly applied to biochar in order to “charge” the biochar with nitrogen prior to applying it to the soil which may be a step of significance importance. Second, a portion of the methane could be used to fire the pyrolysis process at the initial stages in which the process is endothermic (requiring heat and energy) before significant off-gassing when the process become exothermic (giving of heat and energy). I have yet to come across a study which documents the use of pyrolysis and anaerobic methane digesters in such a fashion, but I would imagine the combination would likely be fairly advantageous on a number of fronts.
Larger-scale on-farm methane digester (FarmEnergy.org)
One could imagine designing a holistic agricultural approach that would incorporate biochar production, methane digesters, livestock production, and management of dual-purpose forage and bioenergy crops using low-input high-diversity (LIHD) perennial grasses. Sheep, cattle, goat, dairy, and horse farms could use the manure to generate methane and nitrogen sludge. The livestock could be raised using intensive rotation pasture management, a sustainable production system which is being promoted as a means of carbon sequestration as the growth of perennial pasture grasses can lock up atmospheric carbon in their root structures and regenerate quickly after being grazed. (Research is also being conducted on a garlic supplement which may be able to cut methane emissions of cattle by upwards of 50%.)
Intensive pasture management in combination with methane digesters and biochar production seems to offer a promising avenue of development for farmers (Image courtesy Buggyride.com)
When the livestock are housed in the barn, a good barn designcould allow for efficient collection of manure which could then feed into the anaerobic digester. The methane generated could be used to fire the initial endothermic stages of the pyrolysis reaction, as well as to generate CHP in combination with the syngas stream coming off of the pyrolysis process or used to create nitrogen fertilizer in the Haber-Bosch process. The resulting nitrogen rich sludge from the anaerobic digester could be combined with the biochar produced from the pyrolysis reaction and with composted manure and biomass not used for either the digester or pyrolysis processes creating a powerful slow-releasing soil amendment that could be applied to fields containing perennial forage and energy crops.
Scientific Paper:
Rodriguez et al. (2009) “Effect of biochar and biodigester effluent on growth of maize yields on acidic soil” Livestock Research for Rural Development”
BIOCHAR NO-TILL SYSTEMS
Biochar can be made to work with no-till and conservation tillage methods, however care must be taken so that biochar applications are not prone to wind and water erosion.
Thus mechanical incorporation into the rhizosphere using tillage and disking is a sensible approach, however there is concern that conversion from no-till to tillage practices could lead to a net reduction in soil organic carbon and could offset carbon sequestration gains.
Fortunately, biochar is an extremely stable form of carbon and therefore would not have to be applied on an annual basis. One single large biochar application may, for example, sufficient for 10-20 years or more and thus no-till practices could be used during subsequent years. Or biochar could be incorporated into a conservation tillage regimes (systems which periodically use mechanical tillage) in which biochar could be incorporated once every two or three years as needed.
The Rodale Institute has recently developed an “Organic No-Till” system which utilizes a crop roller that mechanical lays dow the cover crop and crips it to cause die-back in order that the cash crop can be planted in place of the conventional use of herbicides to cause die-back. Periodic tilling of the soil is required and biochar could, therefore, be applied in such a system.
SOIL CARBON MANAGEMENT
Many researchers are examining the potential of soil carbon sequestration as a means of fighting climate change. Much of the research has focused on examining practices such as no-till, conservation tillage, intensive rotational grazing, use of green manures, cover crops, and perennials, mulching, and use of complex crop rotations. While many of these techniques have important benefits beyond the carbon sequestration, quite recent research efforts suggest that the carbon sequestration potential is not quite as high as was originally assumed. This is due to the fact that soils tend to have a maximum carbon holding capacity when–depending on soil type, climate, and agricultural practices–a “carbon saturation” point is reached where carbon gains are offset by carbon losses in the form of CO2 emissions from the soil (Sohi et al., 2009).
Thus, there seems to be an inherent limit–depending on soil type, climate, and agricultural land-use practices–on the maximum carbon sequestration potential we can obtain from soil. So while increasing amounts of organic material can be added such as compost and manure, when the soil reaches the “carbon saturation” point there is a modest amount of organic carbon which can be stabalized into the soil, generally less than 10% of the carbon total carbon input.
The key difference between biochar and the above mentioned soil carbon sequestration practices, in terms of locking up carbon, is that biochar is mainly composed of physically and chemically recalictrant (hard to decompose) materials which can persist for decades, centuries, and even millenia, whereas most other soil carbon amendments are made up of labile (easily decomposed) materials that are mineralized out of the soil upwards of 90% within 5 to 10 years (Lehmann, 2006). Biochar can also be added to soils within reaching “carbon saturation,” the point at which soils can no longer sequester carbon.
Adaptation of Six et al. (2002) carbon saturation or equilibrium. Theoretically, biochar is not subject to this saturation point and therefore could increase total carbon storage capacity of soils. Image courtesy Johannes Lehmann.
In assessing potential gains from biochar soil carbon management, it is not necessary to view biochar in opposition to other sequestration methods–especially considering they may offer added benefits aside from carbon sequestration. Use of perennial and cover crops, for instance, can fix nitrogen, improve soil tilth with tillage and prevent erosion and can also be facilitated and improved with the use of biochar.
BIOCHAR AND POULTRY PRODUCTION
Biochar production from poultry litter is already operational as it offers multiple interlocking benefits. First, biochar offers an effective means of dealing with litter management. Second, the heat produced from biochar production can be used to heat poultry barns during the heating-season thereby offsetting use of conventional fossil fuels. Third, the biochar can be mixed into the litter prior to use in order to prevent loss of valuable nitrogen. This also can lead to improved flock health as it prevents the nitrogenous waste from volatilizing out as ammonia (NH3) which can have negative health implications particularly for the birds’ respiratory and visual system and which can lead to lower laying rates. Fourth, the biochar can be combined with composted poultry litter prior to application to “charge” the biochar with nutrients thereby producing a rich soil amendment. More information on using biochar in poultry production and in other livestock systems can be found in the “Farm” section of the website.
Biochar could be used with poultry production both to heat the barn in addition to an additive to the litter mix to reduce nitrogen volatilization (Agraability Quarterly)
The Frye Farm owned by Mr. Josh Frye in Hardy County, West Virginia has recently installed a gasifier that has been modified to optimize for biochar productionwhich uses none other than the poultry litter to heat the poultry barn. The gasification unit, put in by the Coaltec company, cost approximately $1,000,000 US dollars and is capable of producing 25 to 120 tons of biochar from an annual input of 125 to 600 tons of poultry litter. Frye expects to be able to offset approximately 80-90% of heating requirements using the waste heat from the pyrolysis process to directly heat the poultry barns.
Members of the International Biochar Initiative (IBI) inspecting the biochar produced on the Frye Farm in Hardy County, West Virginia (International Biochar Initiative)
Importantly, Chan et al. (2008) have found that biochars created from poultry litter tend to have more beneficial affectson soil quality and crop production than biochars produced from herbacious, biomass materials.
TERRA PRETA NOVA
Dr. Wim Sombroek(1934-2003), former Secretary General of the International Society of Soil Science who is sometimes given the moniker “the founding father of Terra Preta,” was the first to really call for an active soil management program that would mimick the approach of the Amazonian’s had when they created the “black earth” soils. As a young student of soil science Sombroek visited the Amazon in the mid-1950s and was startled to discover incredibly rich patches of dark black earth. The rich Terra Preta soils him reminded him of the rich black soils of the soils in his country of origin, The Netherlands.
"Founding father" of Terra Preta Wim Sombroek excavating a TP site (Image courtesy of Cornell University)
Plaggen soils are a very rich soil of anthropogenic origin found widely over Western Europe (specifically northwest Germany, Belgium, The Netherlands, Scottland, and Orkney) where carbon-rich peat and sod was used as bedding for livestock thereby soaking up excremental nutrients and was subsequently applied to the agricultural soils in order to proactively improve soil structure and productivity in otherwise unproductive soils (Blume and Leinweber, 2004).
Having been born in The Netherlands in 1934 and lived through War World II and the 1944-1945 The “Hongerwinter” (winter hunger) in which some 18,000 Hollanders died of hunger, Sombroek knew the importance of food and the value of the soil on which it grew to a much greater extent than many of us of ther modern era of plentiful processed food can imagine today. His family persisted on that which they could grow on the small but rich plot of pleggan soil (Charles Mann).
Before Sombroek died in 2003, he called together a team of internationally renowned soil scientists and gave them the challenge of creating a new Terra Preta capable of solving some of the world’s most pressing problems. He called this new Terra Preta, appropriately enough, “Terra Preta Nova.”
Image of plaggen soil common to Western Europe where "plaggen cultivation" was practiced; remarkably similar in appearance and in soil management practices to Terra Preta anthrosols (Image courtesy University of Wisconsin Stevens Point)
The question now is whether it’s possible to meet this challenge Sombroek laid before us. Are we capable of creating rich, self-sustaining soils without doing any further harm to the Earth’s already fragile ecosystems? I would like to believe the answer to this question is yes, yet the real work lies in a) figuring out how to improve soils sustainably based on a bioregional basis, and b) actually doing it on a scale that will make a difference.
BIOCHAR AGROFORESTRY AND AGROECOLOGY
The concept of “forest gardening” has been making a great deal of headway recently as the benefits of perennial crops which typically require far less energy, irrigation, and off-farm fertilizers, are realized. Further, they mimic the patterns and flows natural ecosystems in which synergistic relationships between plant, animal and fungal communities interact and in which nutrients are retained and cycled within the system. Permaculturalist and agroecology practitioners have been at the forefront of advancing the use of perennial cropping systems and forest gardens, but the concept is ancient—perhaps extending back as many as 3,000 years.
Schematic diagram of "stacking" functions within forest garden system (My Urban Garden)
The “stacking” of functions among many diverse levels of the forest (canopy, low tree, bush and bramble, herbaceous, rhizomatic, and climbing vines) offers a three-dimensional productivity as compared to the two-dimensional system of row crops we typically think of when we think of when we think of farming. Thus, the biological richness of a given piece of land being extended spatially upward and temporally all year long (instead of just during the “growing season”) offers unparalled net productivity and minimal to zero tillage.
Forest gardens offer a wide diversity of products that provide a veritable convenience store outside one’s home with a seasonally changing stock of fruits, berries, perennial vegetables, culinary herbs, medicinals, flowers, mushrooms, timber species, coppice stands, fiber species, resins, dies, biofuels, fowl, swine, cattle, ungulates, and wild edibles.
Forest gardens can be practiced in nearly every clime. In my ecotype the range of possible crops includes apples, pears, persimmons, June berries, apricots, cherries, mulberries, quince, jujube, plums, autumn olive, raisin tree, paw paws, chokecherry, elderberry, hazels, chestnuts, pecans, walnuts, hickory, oaks, asparagus, groundnuts, hardy kiwis, grapes, passionflower, currants, gooseberries, strawberries, blue berries, cranberry bush, malva, mint, nettles, mint, daylilies, rhubarb, and many more. An excellent source of native and improved varieties of tree crops for the would-be forest farmer is Oikos Tree Crops. I can also not recommend enough the two volume set entitled “Edible Forest Gardening” by Dave Jacke.
SLASH-AND-CHAR SYSTEMS
“Slash-and-char” has been proposed as an alternative to slash-and-burn agriculture providing a number of ecological, energetic , and economic benefits. Slash-and-char systems could provide a powerful tool for maintaining and improving the sustainability of indigenous agroecological systems in the tropics. A large body of research exists which suggests biochar applications to soil could have multiple beneficial effects, particularly in the tropics or in areas with already low soil quality. Much of the research has been focused on “Terra Preta” (TP) anthrosols in the Amazonian Basin which were likely created by native Ameri-Indians adding large quantities of biochar, in addition to other organic materials, to the soil from ~2000-500 years BP leading to the formation of dark fertile patches of soil corresponding to human settlements.
Slash-and-burn is practiced by some 300 to 500 million people on more than 500 hectares, mostly in the tropics. Systems using this periodic burning technique are often and interchangeably referred to as swidden agriculture, shifting cultivation, slash-and-burn, fire-fallow, and assarting agriculture. Slash-and-burn is common among indigenous and small-holder communities in the humid tropics.
The burning of above-ground biomass has multiple important functions beyond simply clearing land for cultivation. These functions including releasing a pulse of vital nutrients in otherwise highly weathered and nutrient void soil; providing a liming effect to acidic soils; improving cation exchange capacity (CEC); and destroying pathogens, pests, and weed seeds. The widespread use of slash-and-burn systems in the tropics (and historically in temperate climates as well) testifies to the importance of this land management technique.
These indigenous agroecological systems in often consist of highly refined, biologically rich, polycyclic management strategies that are extremely low-cost, low-input and highly sustainable. These systems are capable of providing a significant amount of household nutrients and calories, construction materials, medicines, fodder, fibers, dies, resins, and other useful and/or marketable goods.
For better or worse, slash-and-burn often carries a strong negative connotation. The idea that slash-and-burn agricultural is a highly destructive practice only employed out of desperation by extremely impoverished populations and that is the leading driver of tropical deforestation is typical of the Western perspective. This cultural bias can probably no better be captured but by the 1957 Food and Agriculture Organization (FAO) study that was published in their silviculture publication Unasylva wherein slash-and-burn agriculture is described as, “not only a backward type of agricultural practice. It is also a backward stage of culture in general. In all respects it corresponds to the Neolithic period through which humanity passed between the years 13,000 and 3,000 B.C., considering that the substitution of iron tools for polished stone has made no substantial difference in the way of life.”
Typically these condemnations have typically also been associated with little understanding about the nutrient dynamics or agronomic conditions of the tropics, and less still is known about the highly complex agroecological systems used by indigenous populations who utilize slash-and-burn. Slash-and-burn management is not without problems. However, the wholesale condemnation of an agricultural practice and, indeed, of an entire population of people on Earth as “backward” can be seen in retrospect as a misinformed and culturally defamatory statement of a highly offensive nature. Unfortunately, the hostility to slash-and-burn ignores the salient fact that indigenous peoples the world over have developed notable agroecological models.
While recognizing the importance of indigenous agroecological systems that rely on slash-and-burn, it is also understood that the continuation of slash-and-burn is highly problematic on several fronts. In sum the reasons why slash-and-burn are no longer tenable are that: slash-and-burn extremely difficult to do sustainably considering recent changes in the population, pressure on the forests (particularly from logging companies and “modern” monoculture agriculture exacerbated by increasing biofuel demand), in some cases the over-harvesting of non-timber products (NTFP), and changing precipitation patterns as a result of global climate change which could threaten the forests with massive forest fires possibly transforming them into vast savannahs as they are never able to recover back to a forest state.
Slash-and-burn is no longer sustainable under these new emerging circumstances of population pressure and global climate change. Sanchez and Benites (1987), for instance, have argued that, “Stable alternatives to shifting cultivation are needed for humid tropical locations where increasing demographic pressure no longer permits traditional slash-and-burn agriculture.”
Deforestation is estimated to release approximately 20% of annual anthropogenic emissions at some 2 Gigatons (Gt) per year. The proportion of the anthropogenic emissions associated with slash-and-burn agriculture is difficult to estimate. Adding to the complexity is the fact that many swidden fields are eventually left fallow and allowed regenerate either as a managed system or with natural successional processes, thus mitigating the initial emissions, albeit slowly and often not completely.
In the Amazon, changes to the climate system are expected to cause large-scale shifts in the precipitation patterns resulting in a possible collapse of the rainforest to temperate forests or prairie-savanna regimes. Large-scale and highly destructive fires are also predicted. Malhi et al. (2008) have written that, “The interaction between global climate change and regional deforestation may make Amazonian forests vulnerable to large-scale degradation.”
Slash-and-char has been suggested as a critical alternative to slash-and-burn agriculture. The key change will be to move them from carbon-positive to carbon-negative, thus providing significant benefits to soils and possibly to livelihoods through emerging carbon markets. These benefits, however, will not be automatic and will require significant work to promote small-scale production systems and benefits to local communities instead of focusing exclusively on large-scale biochar producers.
Replacing slash-and-burn agriculture with slash-and-char will likely require additional time and labor costs in addition to training for proper use of kilns and cookstoves. Improved crop production suggests that improved crop production for more fertile soils will partially offset these costs, however, financial incentives for reducing deforestation and sequestering carbon would greatly enhance adaptation and continuous operation.
Slash-and-char systems may be able to work in tandem with Community Based Forestry Management (CBFM). CBFM’s may be able to play an important role in facilitating access to financial incentives for good practice both REDD (Reduced Emissions from Deforestation and Degradation) and biochar soil carbon sequestration credits, thereby passing financial gains to individual indigenous households.
In sum, slash-and-char through pyrolysis proposes to improve the sustainability of agroecology systems in the tropics under rapidly changing global conditions. Slash-and-char will necessarily change certain aspects of biological resource management, but is offered not to replace indigenous knowledge, but to augment it. Indeed, biochar was really only discovered and later pitched by Westerner scientists after having found that indigenous communities in Amazonian and elsewhere had been practicing these techniques for millennia.
Scientific Papers:
Lehmann, Johannes, et al. “Slash-and-Char: A feasible alternative for soil fertility management in the Central Amazon?” Presentation Poster, 2002.
Malhi, Yadvinder, J. Timmons Roberts, Richard A. Betts, Timothy J. Killen, Wenhong Li, Carlos A. Nobre. “Climate Change, Deforestation, and the Fate of the Amazon” Science Magazine; volume 319; 2008.
Peters, Charles M., Alwyn H. Gentry, Robert O. Mendelsohn. “Valuation of an Amazonian Rainforest.” Nature, Vol. 339, 655-656, 1989.
Phillips, Oliver L. et al. “Drought Sensitivity of the Amazon Rainforest.” Science 323, 1344 (2009).
Sanchez, Pedro et al. “Low Input Cropping for Acid Soils of the Humid Tropics.” Science, Vol. 238, 4833, 1521-1527, 1987.
Steiner, Christoph. ”Slash-and-Char as an Alternative to Slash-and-Burn Agriculture.”
REVERSING DESERTIFICATION
As previously mentioned, the United Nations Convention to Combat Desertification (UNCCD) has recently filed a submission with the United Nations Framework Convention on Climate Change (UNFCC) for biochar to be considered as a clean development mechanism for mitigating global climate change, particularly as it applies to arid parts of the world.
Mr. Geoff Lawton, Director of the Permaculture Research Institute of Australia, has demonstrated powerful techniques for “greening the desert” which include Permaculture techniques including use of swales (large ditches dug on contour that aid in water absorption), drip irrigation, planting of nitrogen fixing trees (that also provide shade thereby increasing soil moisture), heavy mulching practices, and use of a diverse variety of perennial crops.
Including biochar within Lawton’s prescription could provide an extremely powerful means of reversing desertification. In most semi-arid and desert climates the soil is nearly void of soil organic carbon (SOC), and thus have the potential to absorb massive quantities of carbon. Generally, the amount of amount of carbon in the soil is a direct indication of soil quality: the greater the amount of SOC, the higher quality the soil. Higher carbon stocks have a direct correlation with increased agricultural yields, higher plant moisture absorption, improved soil tilth, and higher levels of soil biological activity.
Unfortunately, the common practice among many farmers in dryland areas is burning of crop residues which is done to make make cultivation easier, to prevent the spread of disease and pests, and/or it decreases fire risks. Detrimentally, carbon is lost and nutrients quickly exit the system when crop residues are combusted. If instead the farmers produce biochar in biochar cookstoves or to power a local village pyrolysis plants, there is the possibility of improving crop productivity while simultaneously sequestering carbon.
When I really think about the complexity of nature and how everything is connected in the web of life I am still filled with the wonder I held as a young boy. Only difference now is instead of just guessing at how it all works there are other folks doing the legwork to help us all understand the world beneath our feet. One that is every bit as invloved and complex as the world around us and above our heads. So enough of that.
The only real question I walked away from after reading this paper was what is the correct way to determine how to dose Biochar in my garden. Enjoy!!!
P.S. The amount of images exceeds this servers limits. So here is the link where I sourced this paper so you can actually use the pictorials and graphs as it adds an enormous amount of info.
http://biocharfarms.org/farming/
Dr. David A. Laird
USDA National Soil Tilth Laboratory
Biochar not only offers a lot of environmental solutions, it can also provide the farmer and rural communities with a host of real benefits. These include improved soil quality; greater crop yields; higher fertilization efficiency; reduce contamination of groundwater from herbicides and other pollutants; heat for homes, barns, and other applications; and potentially the ability to sell carbon credits and offsets in emerging carbon markets.
If pyrolysis technologies are further developed and refined it may be possible to economically capture energy in the form of syngas and bio-oils that can be used to reduce dependence on foreign oil and gas while reducing carbon emissions.
Biochar could have an even more powerful impact on developing countries where food shortages, population pressures, and declining soil productivity often pose significant challenges. Simply put, biochar production is a long lever from which we can grab a hold of several problems turning them into opportunities to boost efficiency, economics and sustainability for the farmer.
While the potential benefits of biochar are large, there remain a lot of uncertainties and challenges. For instance, while using crop residues for biochar production is appealing, how much can be sustainably harvested without having negative impacts on soil quality? Figuring out means of sustainably harvesting, processing, and shipping biochar without expending too much money or carbon will certainly be a significant challenge. And determining the best methods of applying biochar to fields so that it is not prone to wind and water erosion is also going to be critical.
It’s prudent to stress that while biochar may have strong beneficial impacts on many soils, it’s unlikely that the dramatic increases in crop production reported for highly weathered soils in the tropics will also be shared by already fertile soils in temperate climates.
The most important challenge for biochar, in my opinion, will be integrating it into already existing models of sustainable agriculture that work to improve soil health, ecosystem services, and biodiversity. We will need to find out how biochar can work with other methods of soil carbon sequestration such as no-till, cover cropping, manuring, mulching, and agroforestry.
Biochar farming is likely to be largely experimental during the first decades. Many systems will need to be tried and tested which should offer farmers opportunities for partaking in novel and exciting research trials and a role in developing revolutionary agroecological models of “carbon farming” in which biochar plays a critical role.
BENEFITS OF BIOCHAR APPLICATIONS
Presently, agricultural is one of the most highest greenhouse gas emitting sectors of society. It’s also highly dependent on huge amounts of fossil fuels to run tractors, make fertilizer, and ship food long distances. Biochar offers a unique opportunity to help transform agriculture from being part of the problem to being part of the solution.
There are many questions that need to be addressed when it comes to biochar: What is the best method for producing biochar? What feedstock can or should be used? What is the most efficient way to capture energy from the pyrolysis process? What is the best method to apply biochar to soils and at what rates? Despite the large amount of uncertainty—including possible detrimental effects if not used in an intelligent and appropriate manner—the initial data suggest that the potential benefits of biochar to farming are significant.
Potential benefits that biochar offers for farming include:
1. Improved soil fertility and crop yields
2. Increased fertilizer efficiency use
3. Improved water retention, aeration and soil tilth
4. Higher cation exchange capacity and less nutrient runoff
5. Clean and efficient biomass energy production from crop residues and forest debris
6. Combined heat, power, and refirgeration opportunities from pyrolysis
7. Leads to net sequestration of carbon from the atmosphere to the soil thereby increasing soil organic carbon (SOC)
8. Greater on-farm profitability
9. Can be financed through carbon markets and carbon offsets
10. Decreased nitrous oxide and methane emissions from soils
11. Provides powerful tool for reversing desertification
12. Provides alternative for slash-and-burn agriculture
13. Can work as component of reforestation and aforestation efforts
14. Can produce electricity, bio-oils, and/or hydrogen fuels
15. Can use wide variety of feedstock including crop residues such as wheat and corn straw, poultry litter, cow manure, forest debris, and other farm-based biomass resources
16. Acts as a liming agent to reduce acidity of soils
Biochar is likely to have great variation over climate, soil types, and various agricultural land-use practices, and that many of these figures represent initial findings which require more research in order to substantiate. It is important to note that biochar is likely to have much greater impacts on disturbed, degraded, or high weathered soils than on soils that are high in organic matter content. For instance, a highly weathered soil in the tropics is likely to see much greater impact from biochar application than a fairly fertile soil in a temperate climate. Because most of the research that has taken place in the tropics many of the below listed findings are likely to be much greater than might be observed for the Midwest United States, for instance.
CATION EXCHANGE CAPACITY50% Increase (Glaser, 2002)
FERTILIZER EFFICIENCY 10-30% Increase (Gaunt and Cowie, 2009)
LIMING AGENT 1 Point pH Increase(Lehmann, 2006)
SOIL MOISTURE RETENTION Up to 18% Increase*(Tryon, 1948 )
CROP PRODUCTIVITY 20-120% Increase(Lehman and Rondon, 2006)
METHANE EMISSIONS100% Decrease(Rondon et al., 2005)
NITROUS OXIDE EMISSIONS50% Decrease**(Yanai, 2007; Renner, 2007)
REDUCED BULK DENSITYSoil Dependent(Laird, 2008)
MYCORRHIZAL FUNGI40% Increase***(Warnock, 2007)
BIOLOGICAL NITROGEN FIXATION50-72% Increase(Lehmann and Rondon, 2006)
*Soil moisture retnetion are mostly for sandy soils and could decrease moisture retention in clayey soils. **Nitrous oxide emission have been observed being up to 90%, but other research has found increased emissions under particular circumstances. ***Mycorrhizal fungi have been observed having up to 70% greater population levels with biochar amended soils, but have also been observed to be negatively affected by extremely high biochar application rates.
Scientific Papers:
Gaunt, J; Cowie, A. (2009) “Biochar, Greenhouse Gas Accounting and Emissions Trading” in Lehmann, J.; Joseph, S. “Biochar for Environmental Management: Science and Technology” Earthscan, Sterling, Va.
Glaser, B. Lehmann, J., Steiner, C. Nehls, T., Yousaf, M. and Zech, W. (2002) “Potential of pyrolyzed organic matter in soil amelioration”, in Proceedings of the 12thInternational Soil Conservation Conference, Beijing, China.
Lehmann, J.; John Gaunt; Marco Rondon (2006) “Bio-char Sequestration in Terrestrial Ecosystems” Mitigation and Adaptation Strategies for global Change, 11: 403-427.
BIOCHAR PHYSICAL PROPERTIES
Biochar is a light weight, highly porous material with large surface area. Surface area is important because it affects the soil’s ability to retain nutrients, water, cations, and anions. The high amount of pore space has lead some to describe biochar as an “unerground reef” material that can provide habitat for microorganisms.
BIOCHAR AND CATION EXCHANGE CAPACITY
One of the central benefits of biochar to soil quality and crop yields is its ability to retain key nutrients including nitrogen (N), phosphorus (P), potassium (K), calcium (Ca), and magnesium (Mg), along with other minor and trace nutrients. Increased plant uptake of phophorus, potasium, calcium, zinc (Zn), and copper (Cu) have been observed in the tropics.
The exact characteristics that give biochar the nutreint retention capabilities has been observed in Terra Preta but have yet to be fully explained. The answer likely lies in a combination of i) the physical structure of biochar being highly cavernous and therefore increasing pore space in soils, ii) the chemical property of having a large negatively charged surface area which increases cation exchange capacity (CEC), and iii) the promotion of greater soil organic matter (SOM) levels.
CEC is a measure of the soils ability to retain positively charged “cation” nutrients such as calcium (Ca), magnesium (Mg) potassium (K), and sodium (Na). Basically, CEC is indicates the number of negatively charged sites within a soil where cations can be housed (expressed in terms of milliequivalents of cations per 100 grams of soil).
In general, soils with higher clay contents have higher CEC, whereas soils with principal components of silt or sand have lower CECs. Addition of biochar generally increases CEC, although many studies have found that freshly produced biochar tends to have relatively low CEC and that CEC of biochar improves over period of time which can be quite rapid, perhaps as quickly as a couple of growing seasons.
The exact CEC of a biochar is primarily determined by the feedstock material used and, secondarily, by the pyrolysis conditions the feedstock is subjected to. For instance, biochar derived from poultry litter has been found to have much higher CEC than biochar derived from pine chips under the same pyrolytic conditions. CEC also appears to be maximized under higher treatment temperatures.
There is experimental research that suggests biochar may also improve nitrogen and phosphorus availability and uptake by plants–two critical plant nutrients which are not directly influenced by CEC because they are not positively charged ions. Nitrogen in the form of ammonium ions form associations with the biochar thereby decrease the possibility of nitrogen to be leached from the soil into groundwater or otherwise mineralized out of the soil.
Since nitrogen is often the most expensive and deficient nutrient in many areas of the world (and the number one greenhouse gas emissions source in agriculture), this is a critical benefit. Higher nitrgen retention properties should allow for reduced fertilizer use.
Phosophorus is another major plant nutrient which biochar seems to be able to make more available to plants. Recent warnings that phosphorus may eventually reach peak production suggest that cycling nutrients efficiently through our agricultural, composting, and waste treatment facilities will be vital and that biochar may assist in the process.
In sum, the observation data gathered so far indicates that biochar may have a powerful role in retaining nutrients and thereby increasing nutrient bioavailability, though considerably more observational data is needed.
BIOCHAR AND FERTILIZATION EFFICIENCY
Greater nutrient retention implies that less fertilizers need to be applied to achieve a given crop yield. This is particularly important for nitrogen fertilizer which often constitutes that largest agricultural input with some 207 million metric tons applied in the 2007-2008 growing season globally (FAO, 2008.)
Modeling future demand for nitrogen fertilizer, the Food and Agriculture Organization has predicted that demand will outstrip supply in 2011 at some 240 million metric tons. Further, nitrogen fertilizer use is a significant contributor to global climate change as nitrous oxide (N2O), which is approximately 300 times as powerful a greenhouse gas than carbon dioxide (CO2), is often volatilized into the atmosphere at massive rates.
Because the vast majority of nitrogen fertilizer is derived from natural gas (CH4) via the Haber-Bosch process, the price of nitrogen fertilizer is directly subject to the rises and falls in energy market which although unpredicitable in the short-term are likely to rise under long-term time horizons.
Gaunt and Cowie (2009), in an assessment of biochar’s ability to reduce greenhouse gases, have estimated a 10-30% reduction of nitrogen fertilizer use. Use of biochar has also been observed to reduce emissions of N2O directly from soils by 15-90% (Yanai et al. 2007; Renner, 2007), although one study (Yanai et al. 2007) also found a greater N2O emissions under one set of conditions.
Interestingly, Sohi et al. (2009) have suggested the concept of using syngas from the pyrolysis process to replace natural gas (CH4, methane) to produce nitrogen which could be then be applied to biochar that is produced in the same process to create a powerful carbon and nitrogen rich fertilizer based on the research done by Day et al. (2005). Small scale nitrogen fertilizer plants have been proposed for Africa in order to boost soil fertility and crop production; perhaps it is time we start to consider running these plants using pyrolysis technologies which would vastly increase the sustainability and lower the greenhouse gas emissions associated with producing and applying these nitrogen fertilizers. Below I also examine how anaerobic digester sludge and human urine–both rich in nitrogen–could also be used to charge biochar with nutrients. Use of nitrogen fixing leguminous trees and cover crops in tandem with biochar applications should also be explored.
Scientific Papers:
Day, D., Evans, R.J., Lee, J.W. and Reicosky, D. (2005) “Economical CO2, SOx , and NOx capture from fossil-fuel utilization with combined renewable hydrogen production and large-scale carbon sequestration,” Energy 30, 2558–2579.
Sohi, Saran, Elisa Lopez-Capel, Evelyn Krull, Roland Bol. “Biochar, climate change, and soil: A review to guide future research,” CSIRO 1834-6618, 2009.
Yanai, Y. Toyota, K. and Okazaki, M. (2007) “Effects of charcoal addition on N₂O emissions from soil resulting from rewetting air-dried soil in short-term laboratory experiments”, Soil Science and Plant Nutrition, vol. 53, pp181-188.
BIOCHAR APPLICATION
Many people are interested in applying biochar to their farm or garden which can provide an excellent opportunity for experimenting with and observing the impacts of biochar on crop productivity and soil quality.
The first step is sourcing the biochar material. There are essentially two options: make the charcoal yourself (described later in this section), or buy the charcoal from a company that produces biochar.
The second step is determining the best application methods for your specific area and determining the appropriate rates. “Charging” the biochar with nutrients (such as compost, soluble inorganic fertilizer, or other nutrient sources) has been recommended by many.
An important issue that must be considered is whether biochar should be incorporated using mechanical tillage. Tillage can lead to the loss of large amounts of soil carbon as aeration tends to encourage microbial decomposition of organic matter and subsequent mineralization of soil carbon stocks. A field that has been under no-till management can loss somewhere on the order of 5 or 10 years of carbon accumulation with a single year of tillage. Thus, incorporation of biochar using tillage is counterproductive to our overall goal of soil carbon sequestration. But what if tillage is the only way in some management systems to get the biochar into the ground? Most biochars are likely to be highly susceptible to erosional processes as it is both light (it has a bulk density of 0.17-0.37 tons per cubic meter) and is often hydrophobic when freshly produced. Thus, biochar is extremely prone to runoff from heavy storms with its high buoyancy and it’s also extremely prone to wind erosion due to its light nature and to the fact that biochar tends to have a high dust fraction that can be easily made airborne during transport, incorporation, and post-application.
Because biochar is light and can often contain large dust fractions care must be taken to reduce losses of biochar from the field. The above photo shows loss of biochar during transport. From BlueLeaf Inc., Quebec, Canada.
Major et al. (2009), for instance, found significant loss of biochar (approximately 50%) when applied to tropical soils. Thus, some form of incorporation seems to be necessary. The question is whether and what specific tillage systems are the best or most effective means of incorporation biochar while trying to minimize soil distrubance and loss of soil organic matter and carbon.
Blackwell et al. (2009) provide an excellent description of possible biochar application methods (in “Biochar for Environmental Management” edited by Lehmann and Joseph) including: addition to solid compost with subsequent application via manure spreader; addition of biochar to manure slurry with subsequent spraying onto or injection into soils; pelletization with manure, flour, and water that can be broadcasted onto field; deep banding of biochar using pneumatic systems or incorporation into trenches; and, lastly, top-dressing biochar where biochar is simply spread onto the soil surface without any direct incorporation.
Work must be done to engineer and implement the most efficient and sustainable means of applying biochar for different agricultural systems. At the garden level, incorporation of biochar into a garden plot or raised beds should be a fairly simple task. At the farm scale several alternative tillage systems that may be of interest include the Keyline Plow, organic no-till using a Crop Roller, and the Rotocult.
The Keyline Plow:
The Crop Roller:
Scientific Papers:
Blackwell, P.; Riethmuller, G.; Collins, M. (2009) “Biochar Application to Soil,” in in Lehmann, J.; Joseph, S. (2009) “Biochar for Environmental Management: Science and Technology” Earthscan, Sterling, Va.
Major, J.; Lehmann, J.; Rondon, M.; Goodale, C. (2009) “Fate of Soil Applied Black Carbon,” Global Change Biology.
DOCUMENTING YOUR BIOCHAR EXPERIMENT
I highly recommend to consider running the biochar application as close as possible as a scientific experiment. This can be done by by taking pictures, recording all the pertinent information (e.g. feedstock material and source of biochar, application rate, method of application, crops or pasture grown, any auxiliary fertilizers used, etc.) and documenting the results as carefully as possible. The International Biochar Initiative (IBI) has published a “Guide to Conducting Biochar Trials” by Dr. Julie Major which provides an excellent overview of the steps and procedures for conducting a biochar experiment.
It may even be worthwhile to contact a local university, college, agriculture extension office, or high school in order to get involvement by a member of the scientific community who can assist in conducting a more thorough scientific experiment. The results should be interesting and the process fun. An online database has been established to document biochar trials on the International Biochar Initiative website (contact Dr. Julie Major).
BIOCHAR AND WATER RETENTION
The ability of biochar to improve soil water retention is important in light of global climate change as changing precipitation patterns are predicted with increasing global temperatures. In many areas of the world it is expected that less frequent but more intense storms are likely to become prevalent. This could hurt agricultural production not only because it will likely increase the number of dry days without any precipitation, but when the precipitation does come it will come in more intense storms which risk increasing the erosion of soils. Biochar may have the ability to mitigate drought by increasing soil moisture, while decreasing soil erosion and nutrient leaching. Thus, Micronesia has formally recommended that biochar be considered as a “clean development mechanism” (CDM) within the Kyoto Protocol through the United Nations Convention on Combating Desertification (UNCCD.)
Increased moisture retention and water bioavailability is thought to be a critical factor for greater yields with biochar amended fields. Generally, water retention is increased as the rate of soil organic matter increases. Sohi et al. (2009) have hypothesized that additions of biochar may lead to increased levels of soil organic matter which may partially explain improved water retention, and Glaser et al. (2002) found that Terra Preta soils rich in charcoal had 18% greater water retention than neighboring soils that did not have significant charcoal deposits.
Having a highly porous structure biochar is also thought to improve soil porosity particularly in sandy soils.
Importantly, low temperature pyrolysis biochar have been show to have initial hydrophobic (water avoiding) properties. Thus, research is needed to determine the properties of biochars created by various processes which are important to determine the degree to which they may be able to aid in greater soil water retention and how this property may change over time. For instance, it may be more adventageous to add medium-to-higher temperature pyrolysis biochars to soils that are particularly vulnerable to low soil moisture rates since these biochar are likely to better at retaining soil moisture.
THE SOIL FOOD WEB
The “soil food web” refers to the complex interaction between biological communities of plants, bacteria, protozoa, fungi, nematodes and larger “macro-organisms” which, in part, drive dynamic soil processes. Each of these biological components provides ecosystem services including decomposition, nutrient cycling, filtration and soil formation.
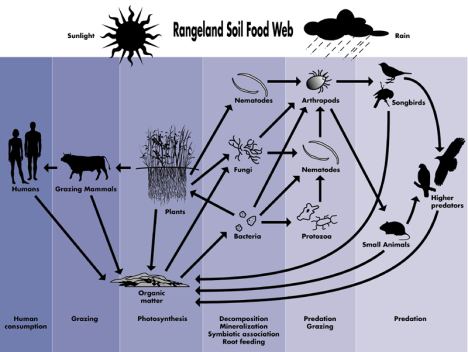
Because biochar influences both physical and chemical properties of soils, it can have a significant effect on the various biological communities. Research on how biochar may alter biological processes in soil is limited, but suggests that biochar could boost population levels of beneficial fungi and bacteria thereby increasing soil health and plant productivity. It has been suggested that biochar with its highly porous exoskeleton is akin to an underground coral reef that could provide habitat, nourishment, and protection to various biological communities in the
MYCORRHIZAL FUNGI
Recent studies suggest that biochar may, under certain circumstances, have a positive affect on the occurence of mycorrhizal fungi populations and their association with plant root systems. Because mycorrhizal fungi are critical and ubiquitous components of terrestrial ecosystems that can have dramatic beneficial effects on plant productivity, there is significant interest in the effect of biochar on this special soil fungi.
Mycorrhizal fungi is an important but often overlooked aspect of soil plant production and soil formation. Mycorrhizal fungi establish mutualistic relationship with the plants. Most often this relationship is symbiotic—both the plant and fungi benefit from one another—but can also be parasitic—the fungi benefits at the detriment of the plan—where extremely low levels of phosphorus or other nutrients are available.
The exchange is basically this: the plant provides energy rich carbohydrates made from photosynthesis to the fungi which the fungi could not otherwise obtain. In exchange the fungi shoots forth a web of hyphae that extend the reach of the plant’s roots and funnel water and nutrients from the soil to the plant. The nutrients delivered to the plants are typically phosphorus, copper, and zinc—the most important being phosphorus. The extent of this relationship is vast with approximately 90% of all terrestrial land plants having associations with mycorrhizal fungi and 66% of terrestrial land plants having associations with the most common soil fungi, arbuscular mycorrhizal fungi (AMF).
Research by Daniel Warnock (see “Mycorrhizal Responses to Biochar in Soils”; Warnock et al., 2007) indicates that the colonization rate of arbuscular mycorrhizal fungi may increase significantly with biochar applications, although later studies using non-herbaceous biochar showed the opposite results (Warnock et al., 2008), therefore highlighting the importance of choosing biochar feedstock carefully and the need for further study to determine the characteristics of biochars from a wide variety of feedstocks produced under multiple pyrolysis procedures. Nishio and Okano (1991) found that when 1 kilogram per square meter of biochar was added to volcanic ash soil that alfalfa associations with AMF increased by 40-80% in Japan.
In 1996, Dr. Sarah F. Wright and PhD student Kristine Nichols, researchers at USDA Agricultural Research Services (ARS), discovered a substance in the soil she later dubbed “glomalin,” a significant and widespread soil carbon source that had previously been completely overlooked. The importance of glomalin has yet to be fully established, however, four characteristics reported by Wright and Nichols make it particularly intriguing.
First, glomalin is pervasive—it is found in practically all soils across the world, though in different magnitudes (more prevalent in temperate regions and less so in tropical areas.) Second, glomalin provides a huge carbon store—roughly roughly 27% of total soil carbon according to ARS research. Third, glomalin lasts a long time in soils—it can survive between 7 and 42 years. And, lastly, it’s sticky—it plays an important role in aggregating and binding together sand, clay, and silt particles which is a precursor to soil formation.
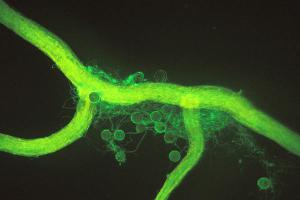
Microscopic image of arbuscular mycorrhizal associations with corn roots; the substance covering the AMF is glomalin thought to be a major source of soil carbon (USDA Agricultural Research Service)
Interestingly, arbuscular mycorrhizal fungi appear to be the only producers of glomalin which occur in abundance on it’s spores and hyphae. Glomalin is a type of glycoprotein (proteins composed of polypeptide and oligosaccharide chains), containing 30 to 40% carbon. Thus glomalin may account for roughly 4 times the amount of total carbon in soil than does humic acid. Wright, speculating on the reason why abuscular mycorrhizal fungi produces glomalin says that, “We’ve seen glomalin on the outside of hyphae, and we believe this is how the hypahae seal themselves so they can carry water and nutrients. It may also be what gives them the rigidity they need to span the air spaces between soil particles.” Wright has speculated that this may partially account for the “missing carbon sink” (see“Glomalin: Hiding Place for One-Third the World’s Carbon”).
Some have speculated that the regenerative nature of glomalin to directly sequester atmospheric carbon from the atmosphere fixing it into the soil may partially explain the “regenerative” nature of ancient Terra Preta soils, though this remains an interesting hypothesis at present with little to no confirmation. Clearly, more research is needed to assess the role of biochar may have on mycorrhizal levels in different soils using various types of biochars.
ARE ALL BIOCHARS THE SAME?
One thing that can be definitively stated about biochar is that they are not all the same. The properties and characteristics (and therefore the agronomic quality) of a particular biochar depends upon a number of interacting feedstock and process variables including the pyrolysis type (slow, fast, intermediate, steam gasification, microwave, etc.); the residence time (fast, slow, intermediate); the temperature (too hot, too cold, just right!); the feedstock material used (all plant material vs. inclusion of manure other high nutrient waste); and whether any additional nutrients were added to the biochar prior to application.
Biochars created from plant materials tend to be lower in nutrient content than biochar created from poultry litter or other manure waste products (Lehmann et al., 2003). One study by Chan et al. (2008) found little to no positive effects to plant growth when a low-nitrogen biochar created solely from plant materials was applied to the soils, but when biochars made from poultry litter (very high in both nitrogen and phosphorus) were applied positive impacts were observed.
(From "A Handful of Carbon" by Johannes Lehmann published in Nature Magazine, May 2007)
All this begs the question, “How is the best biochar made?” “Is there a best pyrolysis method?” “Are there a certain feedstock which are more optimal than others? ” “Is there a specific temperature and residence time at which the biochar obtains optimal properties?” “Is there a certain nutrient rich substance which could best “charge” the biochar prior to application?” We obviously do not have the necessary data to be able to provide answers to all these question. One thing we can be fairly certain of, however, is that the answer to these questions is going to depend the geography, climate, soil types, available materials and desired outcomes of a particular place.
“DESIGNER” BIOCHAR
One might imagine there being a “sweet spot,” so to speak, for pyrolysis production for a region. For instance, if we were in a semi-arid climate we would definitely not want our biochar to have any sort of hydrophobic tendency and therefore might have to stay away from low-temperature pyrolysis. Indeed, we would likely opt for the biochar that delivered the very best water retention qualities possible. We could experiment with the available biomass feedstocks, testing each under a variety of pyrolysis technologies–slow, fast, steam gasification, microwave, etc.–and at different temperature ranges to find which one delivered the very best moisture retention characteristics. In another region, say one with already fairly rich soils, we may not care about the end biochar product nearly as maximizing energy production while simultaneously capturing a certain percentage of carbon as biochar for carbon credits.
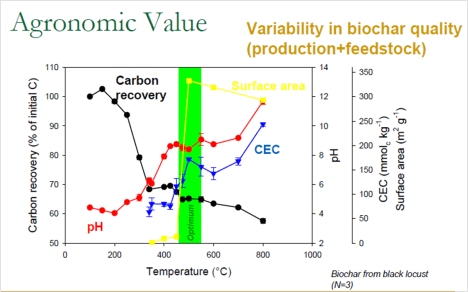
Changing properties of biochar from black locust feedstock as a function of temperature. From Lehmann et al., 2007.
The point of the above example is to demonstrate a) not all biochars are the same, and b) we may be able to use this to our advantage to optimze our production to fit our needs. The most salient point, however, remains that more research is necessary. Hence in a recent publication by Chan et al. (2008), it was stated that, “Despite the recent interest interest in biochars as soil amendments for improving soil quality and increasing soil carbon sequestration, there is inadequate knowledge on the soil amendment properties of these materials produced from different feedstocks and under different pyrolysis conditions.”
Scientific Papers:
Chan, K.Y., Xu, Z. (2009) “Biochar: nutrient Properties and Their Enhancement” in Lehmann, J.; Joseph, S. (2009) “Biochar for Environmental Management: Science and Technology” Earthscan, Sterling, Va.
Chan, K.Y., Van Zwieten, L., Meszaros, I., Downie, A. and Joseph, S. (2007b) “Assessing the agronomic values of constrasting char materials on Australian hardsetting soil”, in Proceedings of the Conference of the International Agrichar Initiative, 30 April-2 May 2007, Terrigal, NSW, Australia.
Novak, J. et al. USDA Agricultural Research Services ongoing research on “Designer Biochars”.
HEALTH AND SAFETY CONCERNS
There are several health and safety issues associated with using biochar including 1) inhalation of charcoal dust during transport and application, 2) safety issues of storing and transporting charcoal mainly from the risk of combustion, 3) introduction of harmful substances into the soil from contaiminated biochar, and 4) use of dangerous and toxic chemicals after biochar application that are adsorped by the charcoal.
A fine charcoal dust often accumulates even when creating large biochar lumps. This can create problems during storage, transport and application as the dust has a tendency to become airborne thereby causing both health issues from inhalation of the dust and safety issues eminating from the possibility that the dust could ignite. Despite the widespread belief that wet charcoal presents safety problems, wetting the biochar will ameliorate health and safety issues eminating from charcoal dust. (If the moistening agent simultaneously charged the biochar with nutrients, this may be an opportunity for accomplishing two tasks at once.) For more information on charcoal handling and storage, see the FAO’s primer on the topic.
One of the principal concerns is the introduction of harmful substances or toxins into the soil. The process of pyrolysis has been found to create both polycylic aromatic hydrocarbons (PAHs) as well as dioxins–both of which are fairly nasty toxins–which may be introduced into the soil with applications of biochar. Intitial studies, however, have found the levels of these potential toxins to be below the safe threshold level.
Sohi et al. (2009) have written that, “Analysis of a limited number of biochar samples has indicated concentrations of toxic combustion products such as polycyclic aromatic hydrocarbons that are not at environmental risk level. However, a more systematic evaluation for a more complete range of other potentially harmful chemical contaminants associated with combustion, as well as toxic substances within feedstocks, has not been made.”
Visual representation of polycycilic aromatic hydrocarbon molecular structure (Wikipedia)
Analyzing fast pyrolysis technologies, Garcia-Perez (2008) found that “PAH production levels are significantly reduced at lower temperatures (below 700°C). In addition, dioxin production is significantly reduced when chlorine and metals are missing.” Gaunt and Lehmann (2008) reported that, “the process temperature parameters under slow pyrolysis are such that we avoid the formation of polyaromatic hydrocarbons in the biochar product.”
Only a handful of studies are available on the issue of PAHs formed during pyrolytic reactions. Sohi et al. (2009) reported that that in personal communication with Dr. David Manning, a Geologist at the University of Newcastle, that recent studies have suggested that the levels of PAHs in biochars are practically the same for most soils. Another analysis by Brown et al. (2006) found PAH concentrations of biochars (3-16 micrograms per gram of biochar) to be lower than that of a controlled burning done within a pine forest (28 micrograms per gram of char).
In order to reduce the risk of producing dioxins and to prevent contaimination of other varieties such as heavy metals precaution should be urged for use of feedstock materials. Sewage sludge, for instance, can be pyrolyzed; however, it would behoove us to abstain from applying the resulting biochar to crops destined for human consumption due to presence of high levels of potentially harmful substances.Thepotential presence of PAHs and dioxins is, in my opinion, the most pernicious issue facing widespread adoption today. A careful, unbaised, and critical examination of this issue is necessary.
Another question that must be addressed is the buildup of secondary compounds. Research has pointed to biochar as being a means to prevent groundwater pollution by capturing fertilizers that lead to eutrophication of waterways as well as pesticides, fungicides, and herbicides such as “atrazine.” (See this recent study by Cao et al. (2009) which documents the ability of biochar derived from dairy manure to trap organic pollutants and heavy metals such as atrazine and lead.)
The ability to capture harmful chemical compounds is typically discussed as a beneficial affect of biochar application, but there remains the question of whether the ability of biochar to capture these compounds may lead to other negative health and safety implications. An novel means of dealing with hydrocarbon compounds has been reported by mycologist Paul Stamets. An interesting research question to test would be whether innoculation of biochar compost piles with fungi such as oyster mushrooms may be able to effectively destroy PAH compounds.
BIOCHAR APPLICATION RATES
The amount of biochar that should be applied to the soil is still an area of active research. Many studies point to 50-60 tons per hectare (20-25 tons per acre) as being an optimal rate of biochar production, however this is a very large amount of material to apply. The objective for biochar farming is probably not going to be applying as much biochar as possible, but rather to achieve the maximize crop production from the most efficient application of biochar. Dr. Johannes Lehmann has reported that, “With relatively small amounts of 2-5 Mg C per hectare [tones/hectare] of bio-char, significant improvements of crop growth can be observed.”
Increase in plant biomass production as a function of amount of biochar applied. From Lehmann and Rondon, 2007.
Thus, the grower would want to know where there area of maximal efficiency lies, which will change according to crop, climate, soil type, etc. This is question of bioregionally appropriate biochar application is an excellent research question for agricultural extension agencies. In addition to trial plots to determine the actual effects, the use of “decision theory” probabilistic modeling would be an appropriate approach to answering this question.
Invariably it is going to be many years—probably decades—before the exact recommended quantities of biochar are worked out for specific crops, for specific soil types, and for specific climates. This is also represents an area that early adopters of biochar farming could greatly benefit the rest of the farming community and the world by converting several acres of their farm to experimental biochar additions. Careful application, observation, and record keeping by farmers–while not necessarily “publishable” in scientific journals–may provide incredibly important initial evidence for the likely effects of biochar in different environments and under a wide variety of conditions.
PURCHASING BIOCHAR
There are a number of companies which have formed recently that are now marketing or selling biochar. The following companies are those which I am aware of that are selling biochar directly to the general public for agricultural uses. Many of them are able to ship amounts ranging from 10 pounds for garden use all the way up to many tons for the larger farm.
A recommendation is that you ask what the process that was used to make the biochar (i.e. slow pyrolysis, fast pyrolysis, or gasification) and what type of feedstock material was used to create the biochar. I would also request to see a copy of a lab analysis which provides information on the contents and characteristics of the biochar including major and minor nutrients, pH, moisture content, and presence of volatiles. It is also a good idea to request that a MSDS (Materials Safety Data Sheet) be provided prior to shipment.
The following is a listing of companies that I am aware of which are presently selling biochar (or natural charcoal without additives marketed for other purposes).
BIOCHAR SUPPLIERS
“CharGrow” from CarbonChar
Requires a minimum of four pallets with each containing 40 50-pound bags (not exactly feasible for anyone except the large land owner.) Based out of New Jersey, US.
“Agri-Char” from BEST Energies
One of the most well established slow pyrolysis companies. Website contains no specific information regarding purchasing of biochar, but may be able to purchase by directly contacting. Based out of Madison, Wisconsin, US and with a test facility in Somersby, Australia.
“EternaGreen™ Biochar” from Biochar Brokers
Biochar produced from woody biomass feedstock; can take larger orders of more than a ton . Based out of Pennsylvania, Colorado, and Tennessee.
“Biochar Plus” from the Biochar Engineering Company
$10US for 10 kg bag of biochar plus . Based out of Golden, Colorado, US.
“Fine Horticulture Charcoal” from Quarter Acres Orchards
“Gallon Bag” (sic) for $6.50 US; I am not knowledgeable whether “carbon negative” pyrolysis is being used to make this charcoal. Based out of Kensington, Maryland.
“Horticultural Charcoal” from Black Gold2 quart bags (sic) available. Sourced from “Western, United States.”
Alibaba Product Search for “Agricultural Charcoal”
Many companies listed from around the world. The sustainability of the companies listed on this site is unknown as are charcoal production methods.
“Hardlump Charcoal” by Cowboy Charcoal Company
A natural hardwood charcoal marketed for barbecuing which can be used as a soil amendment. Claim to have “state-of-the art environmentally compatible wood retort plant.” Based out of Albany, Kentucky.
“Granular Horticulture Charcoal” by Lazarri
Sell several different sizes of charcoal (lump, granular, and powder) from hardwood feedstock coming in 20, 40 and 50 lbs. bags. Based out of Brisbane, California.
“CQuest Biochar” from Dynamotive
Produced in a fast-pyrolysis process with “EcoLogo” Certification; appears to be marketed more as a fuel than a soil amendment; unknown feedstock material; potentially high volatile matter (VM) content. Based out of West Lorne, Ontario, CA.
Various Wood Type Charcoals from Real Montana Charcoal
Sell a wide variety of charcoal from different wood types (apple, maple, plum, oak, etc.); uncertain about sustainability of charring process. Approximately $1/pound for most types. Based out of Florence, Montana, US.
Having listed the following companies from whom you can purchase biochar, I wanted to comment on the issue of shipping biochar long distances: It doesn’t make sense. There’s only a certain range at which biochar can be transported for it 1) to be economical, and 2) to sequester more carbon than is required to make and apply it. There’s a critical threshold distance at which the fact that petroleum is being used to transport the biochar drives the process from being carbon negative to being neutral and then immediately to carbon positive.
I have yet to come across a study which establishes this transportation threshold. The distance is likely to vary somewhat place-to-place, but should be fairly universal within shipping methods (e.g. 18-wheeler tractor trailer, cargo ship, etc.) Fortunately, most areas have ample supplies of biomass materials from which biochar can be produced. The upshot of the fact that biochar can only be economical and sustainable within certain geographic/spatial scales and that in most areas biochar is readily available, is that biochar production probably lends itself toward a smaller-scale, decentralized approach. Fortunately, this is likely to be beneficial in terms of job creation potential, development of local heat and power infrastructure, and promotion of small-to-medium scale operations.
BIOCHAR PRODUCTION FOR GARDEN OR FARM
One of the key benefits of biochar is that it can produced on an extremely small scale although a carefully designed system is necessary to insure that dangerous off-gases are not relased into the atmosphere. For the homegardener there are many designs and descriptions of inexpensive and small-scale methods for making biochar that have been published on the internet. Many of these are based on the use of 55-gallon drums used as retort kilns. The use of the 55-gallon drum retort benefits from its simplicity, inexpensiveness, and ability to produce biochar for the homegarden scale.
A double barrel retort kiln design (Courtesy Twin Oaks Forge)
A serious potential downside of simple charcoal kilns, however, is that they can produce a host of detrimental emissions including CO, NOx and SOx as well as powerful greenhouse gases including methane (CH4) and nitrous oxide (N2O). More elegant 55-gallon retort designs will destroy these gases in a secondary combustion process. If these gases are not combusted the net emissions of biochar production can be detrimental for the climate system (as opposed to being beneficial). A gooddescription of making an effective 55 gallon drum retort that destroys off-gasescan be found on Mr. Folke Gunther’s website. Another 55-gallon drum system I like quite well, pictured above, makes use of two drums which combust the gases directly below the barrels.
A drawback of the 55-gallon drum kiln is that they are not able to produce biochar in the quantity to supply the farm scale. Further, most 55-gallon drum retorts fail to capture or otherwise make use of excess energy produced during the reaction. If there are any heat applications which could be taken advantage of, this would allow for a greater return on the process, hopefully replacing fossil fuel use in the process. For instance, if a heat exchanger were incorporated into the above design design and waste heat was transfered to an insulated hot water tank, the waste heat could be utilized to heat a building space (e.g. greenhouse or poultry barn) with a hydronic steam or hot water system.
Other small-scale farm designs exist. The development of small-scale, high-efficiency pyrolysis cookstoves (such as those being developed by Mr. Robert Flanagan and the WorldStove Company) cleanly produce biochar from crop waste while providing heat for cooking. Development and deployment of these cookstoves could have important implications for least developed countries (LDCs). A 5,000 UK pound ($7,000 US dollar) grant was recently awarded to The Rainforest Information Centre by The Artists Project Planet in order to develop a low-cost, open-source pyrolysis kiln that could be used in developing countries.
A mobile pyrolysis unit well suited for farm-scale use (Courtesy Agri-Therm)
Agri-Therm LLC, based out of Ontario, Canada, is presently working on developing a commercially available fully-automated mobile pyrolysis unit that can process between 2 and 40 tons of biomass per day. Seeing that crop residues and other feedstocks may only be available seasonally it is likely that farmers would only need access to a pyrolysis plant during a portion of the entire year. One could imagine a group of farmers co-investing in one such unit to be used among them instead of having to solely invest in a non-mobile unit.
NOTE ON USING CHARCOAL BRIQUETTES
Briquette charcoals are those which are manufactured to have a uniform size, appearance, and combustion properties. In order to achieve this uniformity a variety of additives are used . These often include starches or clays (as binding agents), sodium or potassium nitrate (to speed up ignition), anthracite coal (to provide longer burn-time), pulverized limestone, and sodium borate (i.e. “borax,” to increase burn time.)
While some of these products (starches, clays, and limestone) would probably be quite benign or even possibly slightly positive to soil quality, the rest would likely be fairly detrimental.
I would recommend against using charcoal briquettes as they contain additives that may be harmful to plants
According to a University of Hawaii study, processed charcoal briquettes can have Volatile Matter (VM) levels as high as 36.4% which could actually decrease plant productivity. Acceptable levels, according to this study, are those below ~7 or 8% VM. In short, I would tend to recommend against using any sort of processed briquette product.
“CHARGING” OF BIOCHAR
After you have acquired the biochar, it is highly recommended that you “charge” the biochar with nutrients before you apply it. This is important: “raw” biochar (straight from the kiln without any nutrient charging) will likely have neutral or even slightly negative effects during the initial phase. This is due to the fact that biochar is very good at holding on to nutrients.
Biochar has a very high cation exchange capacity or CEC and is therefore able to hold onto positively charged nutrients such as calcium (Ca), magnesium (Mg) potassium (K), and sodium (Na), and is also very good at holding onto nitrogen (N) and phosphorus (P). If applied raw to soils nutrients will bond to the biochar thereby making them unavailable for the plant to use (i.e. the “bioavailability” of the nutrients has decreased though the nutrients themselves still remain in the soil.)
If one were to add raw biochar to the soil the long term effect would still be positive, but this would require time for the biochar to “soak up” nutrients until most or all of the pores and negative charges are saturated with nutrients. Then the biochar would act as a slow release fertilizer that efficiently releases a steady stream of nutrients.
Dr. David Laird, of the USDA National Tilth Laboratory, describes biochar as “a fantastic adsorbent and when present in soils it increases the soil’s capacity to adsorb plant nutrients … charcoal contains most of the plant nutrients that were removed when the biomass was harvested and has the capacity to slowly release those nutrients to growing plants,” in a paper on biochar called “The Charcoal Vision“.
Close-up of biochar soil aggregates and mycorrhizal fungal associations on plant roots (Richard Haard)
By pre-charging the biochar with nutrients prior to application we can greatly speed up this absorption process and thereby mitigate any negative effects the biochar may initially have as it tries to satisfy its craving for filling nutrients. Sources of nutrients that can be used with biochar include compost, manure, poultry litter, human urine, and inorganic fertilizers. (This is discussed in more detail below.)
BIOCHAR COMPOSTING
Composting of organic materials is an essential way to cycle nutrients back into the soil especially on organic farms or in areas that don’t have access to chemically derived fertilizers. It then comes at little surprise that Eliot Coleman has said that, “Producing quality compost is the most important job on the organic farm. A lot of the problems I see on farms I visit could be solved by making better compost.” High levels of soil organic matter (SOM) that come from addition of compost, manure, cover crops, and other organic soil builders also decreases the risk of drought by retaining more soil moisture. According to one study by the Rodale Institute, organic fields can often outperform conventional fields by 35-100% during periods of severe drought.
Incorporating biochar into an existing composting system–whether at the garden scale or the farm scale–should not be too difficult and may help retain nutrients that would otherwise be volatilized out of the compost, nitrogen in particular which can escape as ammonia (NH3) gas. Biochar may also likely assist in moisture retention of organic waste piles and increase microbial populations thereby increasing the rate at which materials are broken down into friable compost.
Biochar composting can minimize carbon and nitrogen mineralization and volitalization (Barry Batchelor, Terra Preta List)
Critical to composting is maintaining a balance between carbon (often referred to as “brown” organic materials with high carbon to nitrogen ratios generally above 100:1 such as corn husks, saw dust, and woody mulch) and nitrogen (“green” organic materials with C:N ratios less than 100:1 such as grass clippings, kitchen waste, and leaves) For home gardeners a layering approach of adding consecutive piles of green and brown. “Biodynamics” agricultural practioners often use complex methodologies for creating rich compost involving the addition of rock powders, kelp, and microbial innoculants which I think may provide a nice framework to developing a biochar composting system. For instance, see the following description of making compost. I would imagine including a thick layer of biochar as the foundation of the compost pile would avoid nutrients being leached out, while incorporating thin layers of biochar within the green and brown layers would prevent nutrient volitilization. (More information below on creating “Terra Preta Nova”).
Biochar could be easily incorporated into farm-scale systems such as windrow composting (Virginia Tech Extension Office)
At the farm or muncipal scale biochar could be also be of benefit to composting. The use of biochar within hog slurry retention ponds, windrow composting systems, and manure piles seems quite feasible having the same benefits of reducing the rate of nutrient leaching and volatlization. Use of biochar within poultry production as an addative to the bedding layer also holds a lot of promise (more on that below). I have yet to read any studies of use of biochar within these types of composting systems but there is strong reason to believe that there would be multiple beneficial effects.
BIOCHAR ECOSANITATION
Some may find the idea of using human urine as a fertilizer a disturbing concept. Despite generally being thought of as waste, the fact is that urine is nearly sterile and contains a highly concentrated and relatively well-balanced range of major nutrients with a N:p:K ratio of 18:2:5 as well as a host of minor and micro-nutrients. Using urine as fertilizer could provide a major source of nitrogen particularly for developing countries in which chemically derived nitrogen fertilizers are simply too expensive or altogether unavailable as is the case for much of Africa. The average human excretes approximately 1-2 liters per day, equating to roughly 10 billion liters per day over the entire world or 3.6 trillion liters per year. If , for the sake of argument, one-tenth of this urine were to be effectively captured for use as a nitrogen fertilizer this would equate to some 64 million metric tons (70 million standard tons) thereby offsetting 30% of global nitrogen demand.
Picture of a urine diverting toilet which allows for the separation of dry and liquid human waste
Use of a simple urine diverting toilet, such as the one pictured above, allows for the separation of dry and liquid human waste. Thus urine could be collected, stored and used to charge biochar prior to application.
One of the reasons why urine makes a good fertilizer is that most of it is directly available to plants. Both the potassium (K) and the phosphorus (P) are found in inorganic forms which are readily uptaken by plants. In terms of the nitrogen (N) content, roughly 10-30% of urine is in the form of ammonia and creatine, the remainder being 70-90% in the form of urea. The urea is fairly rapidly decomposition by urease into ammonia and water constituents and subject to volatilization and evaporation. One of the qualities of biochar, however, is to be able to latch onto nitrogen. I have not read any studies on the combined use of biochar and nitrogen, but feel this may be an extremely fruitful area to study in terms of developing sustainable nutrient cycling and fertility programs.
In a 2007 study in Findland, Dr. S. K. Pradhan found that the use of human urine lead to a net increase of 50% more biomass than without urine. Interestingly, it also generated a greater than 9% increase over conventional fertilizers despite the fact that the conventional fertilizers had an N:p:K ratio significantly higher (10.2: 6.8: 19.2) than the urine (10.2: 07: 3.9).
Composting toilets which safely breakdown human feces over a period of time thereby leading to the destruction of pathogens is also recommended. Most manufacturers of composting toilets recommend adding a high carbon material such as peat, sawdust, or wood chips to absorb moisture and prevent odor. Instead, might it be possible and indeed more beneficial to add biochar instead?
Two-part composting toilet that could be used to cycle nutrients in human waste (EconciousMarket)
Being able to cycle nutrients back to the soil is critical for sustainable management of agricultural lands. This is especially the case as we consider the i) the greenhouse gases associated with nitrogen fertilizer manufacturing (which is almost exclusively made from natural gas presently) ii) application of ammonia fertilizer can lead to volatilization of nitrogen in the form of N2O which is 310 times more powerful than CO2 as a greenhouse gas, and iii) fertilizers are likely to become more expensive with the rising cost of natural gas and the declining availability of phosphorus, iv) many communities and municipalities spend upwards of 25% of their energy on sewage treatment, and v) freshwater becomes increasingly scarce (toilets use approximately 30% of household water use.)
This bring new light to the musing Theodore Roosevelt once had that, “Civilized people ought to know how to dispose of the sewage in some better way than putting it in the drinking water.”
TREATMENT WITH ANAEROBIC DIGESTER SLUDGE
Another option for nutrient charging biochar could be done using the byproduct of another energy product system–anaerobic digester sludge. While pyrolysis produces a carbon-rich material that really should be charged with nutrients prior to application, anaerobic digesters produce a nitrogen-rich sludge ideal for charging biochar with nutrients. Further, anaerobic digesters also produce a large stream of methane which could used to fire the initial endothermic stages of slow pyrolysis. Thus biochar production and methane digesters may be well suited to work hand-in-hand.
The Pura Village Community Biogas Project offers a case study and published datafor considering the use of anaerobic digesters with pyrolysis technologies. The project was essentially a cooperative effort in the early 1980’s on behalf of local university researchers, NGOs, and the community of Pura, in Karnataka, India to build a biogas plant to replace wood biomass as their primary fuel for cooking. The sole inputs into the system were water and cow dung. Later the biogas production was fed into a generation system to produce electricity for lighting. Two “floating-drum” digesters were constructed along with a distribution system to provide access to 56 families in the village. Description of a small-scale biogas digester system can be found here.
Anaerobic digesters can range from complex large-scale continuous feed systems to small scale systems constructed of steel drums such as the floating-drum system pictured here (Charlie Forst, 2001)
Based on the performance of the system, the daily input for a ~42.5 m^3 biogas (approximately 60% CH4 and 40% CO2) is 1.25 tons of cattle dung mixed with 1.25 m^3 water per day. The resulting daily output, in addition to the 42.5 m^3 biogas, is approximately 2.45 m^3 of digested slurry containing 3.6 kg of nitrogen (2.2% of the total). The total amount of nitrogen coming out of the biogas system was equal to the amount that was put in—thus, nitrogen is conserved.
Pyrolysis systems could be coupled to biogas systems in a number of ways. First, the nitrogen rich sludge could be directly applied to biochar in order to “charge” the biochar with nitrogen prior to applying it to the soil which may be a step of significance importance. Second, a portion of the methane could be used to fire the pyrolysis process at the initial stages in which the process is endothermic (requiring heat and energy) before significant off-gassing when the process become exothermic (giving of heat and energy). I have yet to come across a study which documents the use of pyrolysis and anaerobic methane digesters in such a fashion, but I would imagine the combination would likely be fairly advantageous on a number of fronts.
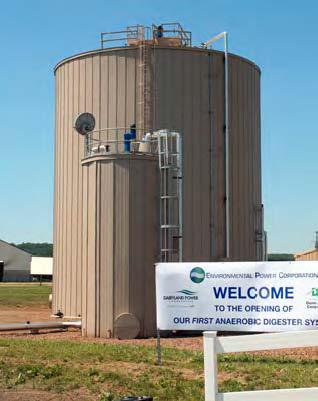
Larger-scale on-farm methane digester (FarmEnergy.org)
One could imagine designing a holistic agricultural approach that would incorporate biochar production, methane digesters, livestock production, and management of dual-purpose forage and bioenergy crops using low-input high-diversity (LIHD) perennial grasses. Sheep, cattle, goat, dairy, and horse farms could use the manure to generate methane and nitrogen sludge. The livestock could be raised using intensive rotation pasture management, a sustainable production system which is being promoted as a means of carbon sequestration as the growth of perennial pasture grasses can lock up atmospheric carbon in their root structures and regenerate quickly after being grazed. (Research is also being conducted on a garlic supplement which may be able to cut methane emissions of cattle by upwards of 50%.)
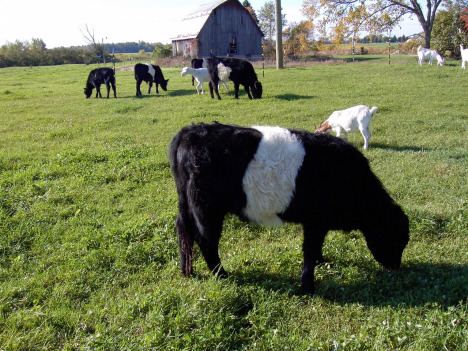
Intensive pasture management in combination with methane digesters and biochar production seems to offer a promising avenue of development for farmers (Image courtesy Buggyride.com)
When the livestock are housed in the barn, a good barn designcould allow for efficient collection of manure which could then feed into the anaerobic digester. The methane generated could be used to fire the initial endothermic stages of the pyrolysis reaction, as well as to generate CHP in combination with the syngas stream coming off of the pyrolysis process or used to create nitrogen fertilizer in the Haber-Bosch process. The resulting nitrogen rich sludge from the anaerobic digester could be combined with the biochar produced from the pyrolysis reaction and with composted manure and biomass not used for either the digester or pyrolysis processes creating a powerful slow-releasing soil amendment that could be applied to fields containing perennial forage and energy crops.
Scientific Paper:
Rodriguez et al. (2009) “Effect of biochar and biodigester effluent on growth of maize yields on acidic soil” Livestock Research for Rural Development”
BIOCHAR NO-TILL SYSTEMS
Biochar can be made to work with no-till and conservation tillage methods, however care must be taken so that biochar applications are not prone to wind and water erosion.
Thus mechanical incorporation into the rhizosphere using tillage and disking is a sensible approach, however there is concern that conversion from no-till to tillage practices could lead to a net reduction in soil organic carbon and could offset carbon sequestration gains.
Fortunately, biochar is an extremely stable form of carbon and therefore would not have to be applied on an annual basis. One single large biochar application may, for example, sufficient for 10-20 years or more and thus no-till practices could be used during subsequent years. Or biochar could be incorporated into a conservation tillage regimes (systems which periodically use mechanical tillage) in which biochar could be incorporated once every two or three years as needed.
The Rodale Institute has recently developed an “Organic No-Till” system which utilizes a crop roller that mechanical lays dow the cover crop and crips it to cause die-back in order that the cash crop can be planted in place of the conventional use of herbicides to cause die-back. Periodic tilling of the soil is required and biochar could, therefore, be applied in such a system.
SOIL CARBON MANAGEMENT
Many researchers are examining the potential of soil carbon sequestration as a means of fighting climate change. Much of the research has focused on examining practices such as no-till, conservation tillage, intensive rotational grazing, use of green manures, cover crops, and perennials, mulching, and use of complex crop rotations. While many of these techniques have important benefits beyond the carbon sequestration, quite recent research efforts suggest that the carbon sequestration potential is not quite as high as was originally assumed. This is due to the fact that soils tend to have a maximum carbon holding capacity when–depending on soil type, climate, and agricultural practices–a “carbon saturation” point is reached where carbon gains are offset by carbon losses in the form of CO2 emissions from the soil (Sohi et al., 2009).
Thus, there seems to be an inherent limit–depending on soil type, climate, and agricultural land-use practices–on the maximum carbon sequestration potential we can obtain from soil. So while increasing amounts of organic material can be added such as compost and manure, when the soil reaches the “carbon saturation” point there is a modest amount of organic carbon which can be stabalized into the soil, generally less than 10% of the carbon total carbon input.
The key difference between biochar and the above mentioned soil carbon sequestration practices, in terms of locking up carbon, is that biochar is mainly composed of physically and chemically recalictrant (hard to decompose) materials which can persist for decades, centuries, and even millenia, whereas most other soil carbon amendments are made up of labile (easily decomposed) materials that are mineralized out of the soil upwards of 90% within 5 to 10 years (Lehmann, 2006). Biochar can also be added to soils within reaching “carbon saturation,” the point at which soils can no longer sequester carbon.
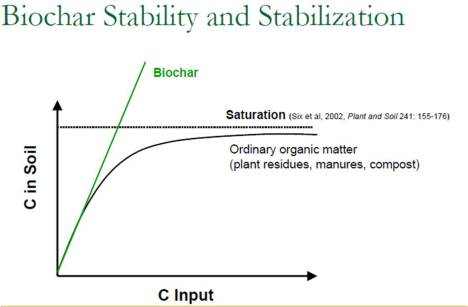
Adaptation of Six et al. (2002) carbon saturation or equilibrium. Theoretically, biochar is not subject to this saturation point and therefore could increase total carbon storage capacity of soils. Image courtesy Johannes Lehmann.
In assessing potential gains from biochar soil carbon management, it is not necessary to view biochar in opposition to other sequestration methods–especially considering they may offer added benefits aside from carbon sequestration. Use of perennial and cover crops, for instance, can fix nitrogen, improve soil tilth with tillage and prevent erosion and can also be facilitated and improved with the use of biochar.
BIOCHAR AND POULTRY PRODUCTION
Biochar production from poultry litter is already operational as it offers multiple interlocking benefits. First, biochar offers an effective means of dealing with litter management. Second, the heat produced from biochar production can be used to heat poultry barns during the heating-season thereby offsetting use of conventional fossil fuels. Third, the biochar can be mixed into the litter prior to use in order to prevent loss of valuable nitrogen. This also can lead to improved flock health as it prevents the nitrogenous waste from volatilizing out as ammonia (NH3) which can have negative health implications particularly for the birds’ respiratory and visual system and which can lead to lower laying rates. Fourth, the biochar can be combined with composted poultry litter prior to application to “charge” the biochar with nutrients thereby producing a rich soil amendment. More information on using biochar in poultry production and in other livestock systems can be found in the “Farm” section of the website.
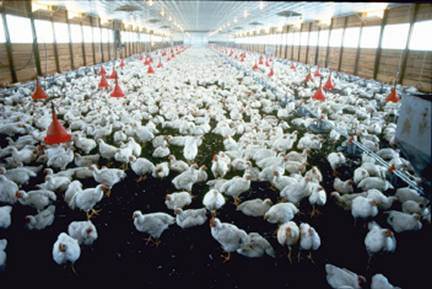
Biochar could be used with poultry production both to heat the barn in addition to an additive to the litter mix to reduce nitrogen volatilization (Agraability Quarterly)
The Frye Farm owned by Mr. Josh Frye in Hardy County, West Virginia has recently installed a gasifier that has been modified to optimize for biochar productionwhich uses none other than the poultry litter to heat the poultry barn. The gasification unit, put in by the Coaltec company, cost approximately $1,000,000 US dollars and is capable of producing 25 to 120 tons of biochar from an annual input of 125 to 600 tons of poultry litter. Frye expects to be able to offset approximately 80-90% of heating requirements using the waste heat from the pyrolysis process to directly heat the poultry barns.
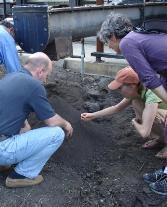
Members of the International Biochar Initiative (IBI) inspecting the biochar produced on the Frye Farm in Hardy County, West Virginia (International Biochar Initiative)
Importantly, Chan et al. (2008) have found that biochars created from poultry litter tend to have more beneficial affectson soil quality and crop production than biochars produced from herbacious, biomass materials.
TERRA PRETA NOVA
Dr. Wim Sombroek(1934-2003), former Secretary General of the International Society of Soil Science who is sometimes given the moniker “the founding father of Terra Preta,” was the first to really call for an active soil management program that would mimick the approach of the Amazonian’s had when they created the “black earth” soils. As a young student of soil science Sombroek visited the Amazon in the mid-1950s and was startled to discover incredibly rich patches of dark black earth. The rich Terra Preta soils him reminded him of the rich black soils of the soils in his country of origin, The Netherlands.
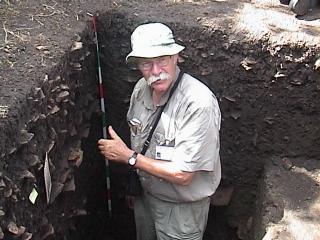
"Founding father" of Terra Preta Wim Sombroek excavating a TP site (Image courtesy of Cornell University)
Plaggen soils are a very rich soil of anthropogenic origin found widely over Western Europe (specifically northwest Germany, Belgium, The Netherlands, Scottland, and Orkney) where carbon-rich peat and sod was used as bedding for livestock thereby soaking up excremental nutrients and was subsequently applied to the agricultural soils in order to proactively improve soil structure and productivity in otherwise unproductive soils (Blume and Leinweber, 2004).
Having been born in The Netherlands in 1934 and lived through War World II and the 1944-1945 The “Hongerwinter” (winter hunger) in which some 18,000 Hollanders died of hunger, Sombroek knew the importance of food and the value of the soil on which it grew to a much greater extent than many of us of ther modern era of plentiful processed food can imagine today. His family persisted on that which they could grow on the small but rich plot of pleggan soil (Charles Mann).
Before Sombroek died in 2003, he called together a team of internationally renowned soil scientists and gave them the challenge of creating a new Terra Preta capable of solving some of the world’s most pressing problems. He called this new Terra Preta, appropriately enough, “Terra Preta Nova.”
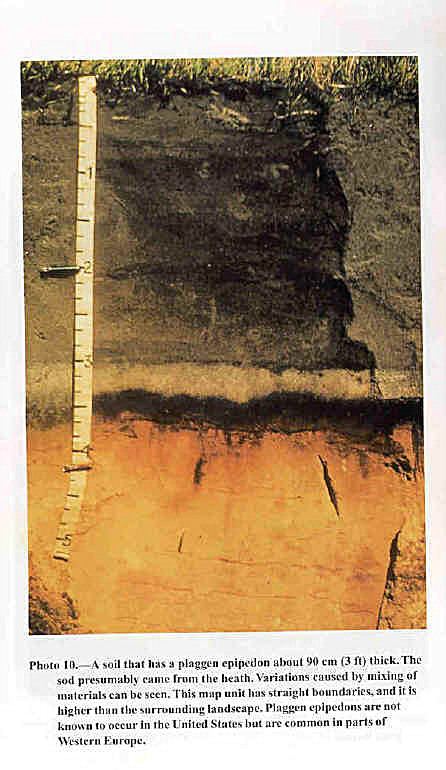
Image of plaggen soil common to Western Europe where "plaggen cultivation" was practiced; remarkably similar in appearance and in soil management practices to Terra Preta anthrosols (Image courtesy University of Wisconsin Stevens Point)
The question now is whether it’s possible to meet this challenge Sombroek laid before us. Are we capable of creating rich, self-sustaining soils without doing any further harm to the Earth’s already fragile ecosystems? I would like to believe the answer to this question is yes, yet the real work lies in a) figuring out how to improve soils sustainably based on a bioregional basis, and b) actually doing it on a scale that will make a difference.
BIOCHAR AGROFORESTRY AND AGROECOLOGY
The concept of “forest gardening” has been making a great deal of headway recently as the benefits of perennial crops which typically require far less energy, irrigation, and off-farm fertilizers, are realized. Further, they mimic the patterns and flows natural ecosystems in which synergistic relationships between plant, animal and fungal communities interact and in which nutrients are retained and cycled within the system. Permaculturalist and agroecology practitioners have been at the forefront of advancing the use of perennial cropping systems and forest gardens, but the concept is ancient—perhaps extending back as many as 3,000 years.
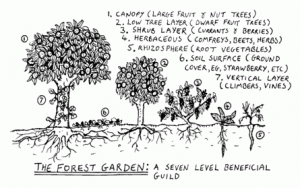
Schematic diagram of "stacking" functions within forest garden system (My Urban Garden)
The “stacking” of functions among many diverse levels of the forest (canopy, low tree, bush and bramble, herbaceous, rhizomatic, and climbing vines) offers a three-dimensional productivity as compared to the two-dimensional system of row crops we typically think of when we think of when we think of farming. Thus, the biological richness of a given piece of land being extended spatially upward and temporally all year long (instead of just during the “growing season”) offers unparalled net productivity and minimal to zero tillage.
Forest gardens offer a wide diversity of products that provide a veritable convenience store outside one’s home with a seasonally changing stock of fruits, berries, perennial vegetables, culinary herbs, medicinals, flowers, mushrooms, timber species, coppice stands, fiber species, resins, dies, biofuels, fowl, swine, cattle, ungulates, and wild edibles.
Forest gardens can be practiced in nearly every clime. In my ecotype the range of possible crops includes apples, pears, persimmons, June berries, apricots, cherries, mulberries, quince, jujube, plums, autumn olive, raisin tree, paw paws, chokecherry, elderberry, hazels, chestnuts, pecans, walnuts, hickory, oaks, asparagus, groundnuts, hardy kiwis, grapes, passionflower, currants, gooseberries, strawberries, blue berries, cranberry bush, malva, mint, nettles, mint, daylilies, rhubarb, and many more. An excellent source of native and improved varieties of tree crops for the would-be forest farmer is Oikos Tree Crops. I can also not recommend enough the two volume set entitled “Edible Forest Gardening” by Dave Jacke.
SLASH-AND-CHAR SYSTEMS
“Slash-and-char” has been proposed as an alternative to slash-and-burn agriculture providing a number of ecological, energetic , and economic benefits. Slash-and-char systems could provide a powerful tool for maintaining and improving the sustainability of indigenous agroecological systems in the tropics. A large body of research exists which suggests biochar applications to soil could have multiple beneficial effects, particularly in the tropics or in areas with already low soil quality. Much of the research has been focused on “Terra Preta” (TP) anthrosols in the Amazonian Basin which were likely created by native Ameri-Indians adding large quantities of biochar, in addition to other organic materials, to the soil from ~2000-500 years BP leading to the formation of dark fertile patches of soil corresponding to human settlements.
Slash-and-burn is practiced by some 300 to 500 million people on more than 500 hectares, mostly in the tropics. Systems using this periodic burning technique are often and interchangeably referred to as swidden agriculture, shifting cultivation, slash-and-burn, fire-fallow, and assarting agriculture. Slash-and-burn is common among indigenous and small-holder communities in the humid tropics.
The burning of above-ground biomass has multiple important functions beyond simply clearing land for cultivation. These functions including releasing a pulse of vital nutrients in otherwise highly weathered and nutrient void soil; providing a liming effect to acidic soils; improving cation exchange capacity (CEC); and destroying pathogens, pests, and weed seeds. The widespread use of slash-and-burn systems in the tropics (and historically in temperate climates as well) testifies to the importance of this land management technique.
These indigenous agroecological systems in often consist of highly refined, biologically rich, polycyclic management strategies that are extremely low-cost, low-input and highly sustainable. These systems are capable of providing a significant amount of household nutrients and calories, construction materials, medicines, fodder, fibers, dies, resins, and other useful and/or marketable goods.
For better or worse, slash-and-burn often carries a strong negative connotation. The idea that slash-and-burn agricultural is a highly destructive practice only employed out of desperation by extremely impoverished populations and that is the leading driver of tropical deforestation is typical of the Western perspective. This cultural bias can probably no better be captured but by the 1957 Food and Agriculture Organization (FAO) study that was published in their silviculture publication Unasylva wherein slash-and-burn agriculture is described as, “not only a backward type of agricultural practice. It is also a backward stage of culture in general. In all respects it corresponds to the Neolithic period through which humanity passed between the years 13,000 and 3,000 B.C., considering that the substitution of iron tools for polished stone has made no substantial difference in the way of life.”
Typically these condemnations have typically also been associated with little understanding about the nutrient dynamics or agronomic conditions of the tropics, and less still is known about the highly complex agroecological systems used by indigenous populations who utilize slash-and-burn. Slash-and-burn management is not without problems. However, the wholesale condemnation of an agricultural practice and, indeed, of an entire population of people on Earth as “backward” can be seen in retrospect as a misinformed and culturally defamatory statement of a highly offensive nature. Unfortunately, the hostility to slash-and-burn ignores the salient fact that indigenous peoples the world over have developed notable agroecological models.
While recognizing the importance of indigenous agroecological systems that rely on slash-and-burn, it is also understood that the continuation of slash-and-burn is highly problematic on several fronts. In sum the reasons why slash-and-burn are no longer tenable are that: slash-and-burn extremely difficult to do sustainably considering recent changes in the population, pressure on the forests (particularly from logging companies and “modern” monoculture agriculture exacerbated by increasing biofuel demand), in some cases the over-harvesting of non-timber products (NTFP), and changing precipitation patterns as a result of global climate change which could threaten the forests with massive forest fires possibly transforming them into vast savannahs as they are never able to recover back to a forest state.
Slash-and-burn is no longer sustainable under these new emerging circumstances of population pressure and global climate change. Sanchez and Benites (1987), for instance, have argued that, “Stable alternatives to shifting cultivation are needed for humid tropical locations where increasing demographic pressure no longer permits traditional slash-and-burn agriculture.”
Deforestation is estimated to release approximately 20% of annual anthropogenic emissions at some 2 Gigatons (Gt) per year. The proportion of the anthropogenic emissions associated with slash-and-burn agriculture is difficult to estimate. Adding to the complexity is the fact that many swidden fields are eventually left fallow and allowed regenerate either as a managed system or with natural successional processes, thus mitigating the initial emissions, albeit slowly and often not completely.
In the Amazon, changes to the climate system are expected to cause large-scale shifts in the precipitation patterns resulting in a possible collapse of the rainforest to temperate forests or prairie-savanna regimes. Large-scale and highly destructive fires are also predicted. Malhi et al. (2008) have written that, “The interaction between global climate change and regional deforestation may make Amazonian forests vulnerable to large-scale degradation.”
Slash-and-char has been suggested as a critical alternative to slash-and-burn agriculture. The key change will be to move them from carbon-positive to carbon-negative, thus providing significant benefits to soils and possibly to livelihoods through emerging carbon markets. These benefits, however, will not be automatic and will require significant work to promote small-scale production systems and benefits to local communities instead of focusing exclusively on large-scale biochar producers.
Replacing slash-and-burn agriculture with slash-and-char will likely require additional time and labor costs in addition to training for proper use of kilns and cookstoves. Improved crop production suggests that improved crop production for more fertile soils will partially offset these costs, however, financial incentives for reducing deforestation and sequestering carbon would greatly enhance adaptation and continuous operation.
Slash-and-char systems may be able to work in tandem with Community Based Forestry Management (CBFM). CBFM’s may be able to play an important role in facilitating access to financial incentives for good practice both REDD (Reduced Emissions from Deforestation and Degradation) and biochar soil carbon sequestration credits, thereby passing financial gains to individual indigenous households.
In sum, slash-and-char through pyrolysis proposes to improve the sustainability of agroecology systems in the tropics under rapidly changing global conditions. Slash-and-char will necessarily change certain aspects of biological resource management, but is offered not to replace indigenous knowledge, but to augment it. Indeed, biochar was really only discovered and later pitched by Westerner scientists after having found that indigenous communities in Amazonian and elsewhere had been practicing these techniques for millennia.
Scientific Papers:
Lehmann, Johannes, et al. “Slash-and-Char: A feasible alternative for soil fertility management in the Central Amazon?” Presentation Poster, 2002.
Malhi, Yadvinder, J. Timmons Roberts, Richard A. Betts, Timothy J. Killen, Wenhong Li, Carlos A. Nobre. “Climate Change, Deforestation, and the Fate of the Amazon” Science Magazine; volume 319; 2008.
Peters, Charles M., Alwyn H. Gentry, Robert O. Mendelsohn. “Valuation of an Amazonian Rainforest.” Nature, Vol. 339, 655-656, 1989.
Phillips, Oliver L. et al. “Drought Sensitivity of the Amazon Rainforest.” Science 323, 1344 (2009).
Sanchez, Pedro et al. “Low Input Cropping for Acid Soils of the Humid Tropics.” Science, Vol. 238, 4833, 1521-1527, 1987.
Steiner, Christoph. ”Slash-and-Char as an Alternative to Slash-and-Burn Agriculture.”
REVERSING DESERTIFICATION
As previously mentioned, the United Nations Convention to Combat Desertification (UNCCD) has recently filed a submission with the United Nations Framework Convention on Climate Change (UNFCC) for biochar to be considered as a clean development mechanism for mitigating global climate change, particularly as it applies to arid parts of the world.
Mr. Geoff Lawton, Director of the Permaculture Research Institute of Australia, has demonstrated powerful techniques for “greening the desert” which include Permaculture techniques including use of swales (large ditches dug on contour that aid in water absorption), drip irrigation, planting of nitrogen fixing trees (that also provide shade thereby increasing soil moisture), heavy mulching practices, and use of a diverse variety of perennial crops.
Including biochar within Lawton’s prescription could provide an extremely powerful means of reversing desertification. In most semi-arid and desert climates the soil is nearly void of soil organic carbon (SOC), and thus have the potential to absorb massive quantities of carbon. Generally, the amount of amount of carbon in the soil is a direct indication of soil quality: the greater the amount of SOC, the higher quality the soil. Higher carbon stocks have a direct correlation with increased agricultural yields, higher plant moisture absorption, improved soil tilth, and higher levels of soil biological activity.
Unfortunately, the common practice among many farmers in dryland areas is burning of crop residues which is done to make make cultivation easier, to prevent the spread of disease and pests, and/or it decreases fire risks. Detrimentally, carbon is lost and nutrients quickly exit the system when crop residues are combusted. If instead the farmers produce biochar in biochar cookstoves or to power a local village pyrolysis plants, there is the possibility of improving crop productivity while simultaneously sequestering carbon.